
Unit 1 Introduction
This unit serves as an introduction to the basic biology of the brain and is required for understanding subsequent units. Therefore, this Neurons & Brain Anatomy unit should be covered after the launch lesson and before diving into any other topics from this curriculum.
This unit covers some of the basic biology of the brain and is broken into two main sections: Cells of the Brain and Brain Anatomy. Section 1 will discuss what neurons are and how these cells allow information to be transmitted all the way from a finger (where you just got poked) to many different parts of the brain (that allow you to feel the pain and generate an “ouch” response)? Section 1 also presents neurotransmission, or how neurons send chemical signals to other neurons, which will be relevant in Unit 5 when covering substance use and addiction. Section 2 covers questions like what are the parts of the brain, what do they do, and how do they compare to the brains of other animals will be covered to build a foundation of understanding for students.
What's In This Unit?
Section 1: Cells of the Brain
- Lesson 1: Neurons
- Lesson 2: Action Potentials
- Lesson 3: Synaptic Transmission
Section 2: Brain Anatomy
- Lesson 4: Lesion Studies
- Lesson 5: Parts and Regions of the Brain
- Lesson 6: Comparative Neuroanatomy
Terms & Definitions: Unit 1
- Neuron – Special type of cell in the body that has a unique structure (containing an axon and dendrites) and which transmits signals throughout the nervous system.
- Glia – Another type of cell in the nervous system which does not transmit signals the same way that neurons do but plays many other important roles in the brain.
- Axon – The part of a neuron that sends a signal on to the next neuron. Neurons only have one axon each, but this axon can branch into many axon terminals that connect to different neurons.
- Action Potential – An electrical signal that travels down the length of a neuron’s axon.
- Dendrite – The part of a neuron that receives incoming signals. Neurons can have multiple dendrites.
- Myelin – A fatty coating that wraps around axons. This insulates the axon and allows the signal to travel faster and farther.
- Synaptic Cleft – The region in between the axon terminal of the sending neuron and the dendrite of the receiving neuron. Neurotransmitters are released into this cleft to pass information between the neurons.
- Synapse - The functional site of connection between two neurons, consisting of the synaptic cleft and the cell membranes on either side.
- Neurotransmitters – The chemical messengers used to transmit information from one neuron to another. There are over 100 identified types of neurotransmitters (e.g. serotonin, dopamine, GABA). They are released from compartments called vesicles in the sending neuron and bind to receptors in the receiving neuron.
- Lesion – Damage to a region of the brain. This can occur through injury, disease. In other animals, researchers can induce lesions for experimental purposes.
- Gray matter - Brain tissue made up of neuron cell bodies and dendrites, as well as some types of glia.
- White matter - Brain tissue made up of the myelinated axons of neurons (as well as the glial cells that provide the myelin sheath).
- Cerebral cortex - The wrinkly outer layer on the surface of the brain where most higher order complex thinking, or cognition, takes place.
- Frontal lobe - Area of cerebral cortex associated with decision-making, planning, judgment, and motor control.
- Parietal lobe - Area of cerebral cortex which processes sensory and spatial information and integrates information coming from different senses.
- Temporal lobe - Area of cerebral cortex which includes regions responsible for sound processing, language comprehension, and memory formation.
- Occipital lobe - Area of cerebral cortex which is primarily responsible for processing visual information, including color and motion detection.
- Cerebellum - A brain region involved in the coordination of complex movements.
- Brain stem - The part of the brain connecting it to the spinal cord, important for controlling automatic functions of the body like heart rate, sleep cycles, and breathing.
- Corpus callosum - A region of white matter tracts that connects the left and right hemispheres.
- Amygdala - A brain region involved with emotional response, including fear and motivation.
- Hippocampus - A brain region that plays an important role in forming long-term memories.
Section 1: Cells of the Brain
Lesson 1: Neurons
Objective: Students will be able to explain how the structure of neurons allows them to send and receive signals.
ENGAGE/HOOK: Cell Comparison (10 min)
To introduce this lesson, show students the pictures below of a pancreatic cell on the left and a neuron on the right, and ask:
- What do you think these diagrams represent? Have you seen these types of diagrams before?
- What do you think this lesson will be about based on these diagrams?
Image
Both these cells come from the human body and both contain standard organelles, including a nucleus. However, neurons are more complex than many other types of body cells, featuring dendrites and axons by which they can form connections with other cells. Image credit: BioRender.com
Once students have shared out their thoughts on the cells, guide them through an introductory discussion:
- Explain to students that this lesson will be all about neurons: the cells of the nervous system that transmit signals throughout the body. Ask students:
- What do you observe about the two cells?
- How are they similar, and how are they different?
- Once students have a chance to discuss what they observe explain the following:
- The goal is to see the differences in structure. Most cells in the human body, like this pancreatic cell (an acinar cell), do not extend long distances to transmit messages. This is reflected in the compact structure of the cell, compared to the long axon found in neurons like the one shown here. The left cell is a cell from the pancreas that produces digestive enzymes. The right cell is a special cell that passes information throughout the body: a nerve cell, or neuron. Point out the three unique parts of the neuron: the dendrites, the cell body, and the axon. Explain that the dendrites receive information, and axons send it, and that the cell body does all the normal functions that would occur in any common animal cell, containing all the usual organelles including the nucleus.
EXPLORE: Build a Network (30 min)
Guide students through the use of this interactive simulation of neural signaling using the steps below.
- Have students navigate to the simulation website.
- Ask students to explore how neurons connect and send signals by moving neurons around and watching signals move.
- Ask students to click the “science behind it” button and read about neurons.
- Challenge students to build networks with different types of connectivity, e.g. a feedback loop or signal amplification through multiple branches.
- After completing the interactive ask students to reflect using the questions below:
- What parts of neurons connect to transmit a signal?
- Can a neuron receive signals from multiple neurons? Can it send signals to multiple neurons? Why does this connectivity matter?
- Sending a signal from one neuron to the next is like a switch—it either happens or it doesn’t. Why might that be important to the brain’s communication system?
- What network structure would you design to turn a small input into a strong signal?
EXPLAIN: Neurons and Glia
The following information is teacher-facing and can be utilized to teach students new information in whatever format works best for you and your students.
Key Points:
- Neurons and glia are cells that make up the nervous system.
- There are approximately 86 billion neurons in the brain and are likely a similar number of glia, the support cells.
- Neurons have the unique ability to be electrically excited, so they can transmit signals very quickly throughout the body.
- Neurons have dendrites, which receive signals, and axons, which send signals.
- Glia are the support cells of the brain and there are several different types that each play a specific role
Neurons
It is difficult to grasp really large numbers, so when discussing how many neurons the average person has in their brain, people often compare it to the number of stars in our galaxy. However, the Milky Way has more stars (over 200 billion) than you have neurons in your head (around 86 billion—still large but not as large). Neurons have the important job of encoding and transmitting information all over the brain and the body, bringing information from the outside world to the brain, and sending information from the brain out to the body again. Neurons send information down their long axons encoded as electrical signals. There are all kinds of neurons that come in all shapes and sizes, usually related to their functions (like the examples in the illustration below). Neurons that receive a lot of information will have a large set of dendrites, called a dendritic arbor (since they often are tree-like in appearance) and a short or small axon. In contrast, neurons that are mostly for sending information may have few dendrites and a very large, long axon.
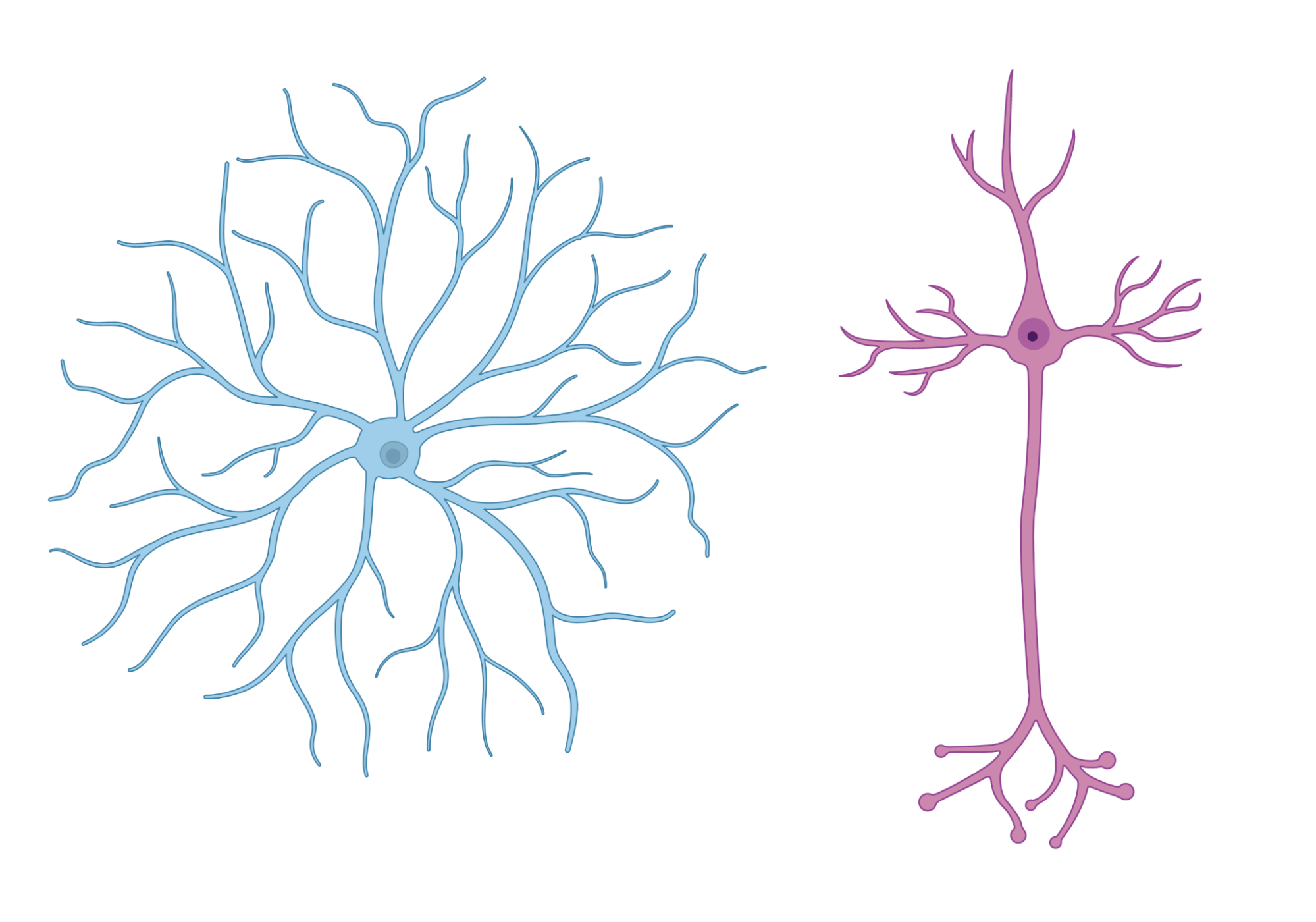
Some neurons have a small axon or no axon at all. Other neurons are defined by one long central axon that can extend up to several feet in length. Image credit: BioRender.com
Glia
Glia are often referred to as the support cells of the brain. There are several different types of glia, each with their own roles to play. Some glia act as immune cells for the brain, keeping infections at bay, other’s help nourish neurons and keep their communication running smoothly. Others form layers of insulation (called myelin, explored further in Lesson 3) around the axons of some types of neurons, allowing the neuron’s electrical signals to move more quickly and efficiently. There has been some debate over the years regarding how many glia are found in the brain; modern techniques point to a similar number of glia and neurons.
Additional Resources
- This website offers an introduction to neurons and action potentials. (University of Queensland)
- This website has some basic facts about neurons and also demonstrates one way in which neurons can be categorized. (Neuroscience for Kids)
- See helpful images and descriptions of the parts of a neuron. (Boundless Biology)
ELABORATE: Motor Neuron Model (30 min)
Have students build a neuron with playdough or modeling clay, using different colors to represent the different parts: soma (cell body), nucleus, dendrites, axon and axon terminals.
Use this Build a Neuron activity from Indiana University as a guide. Abbreviated steps are below.
Note: This activity has them add myelin sheath and neurotransmitters which have not yet been covered in this lesson, so you could skip those as a modification.
- Make a ball out of playdough and flatten into a circle. Lay the disk on the table to represent the soma, or cell body.
- Make a smaller ball of another color and smash into the disk to represent the nucleus.
- Roll-out logs and create tree-like branches to represent dendrites.
- Attach a long log to the cell body to represent the axon.
- Add smaller branches at the end of the “axon” to show axon terminals.
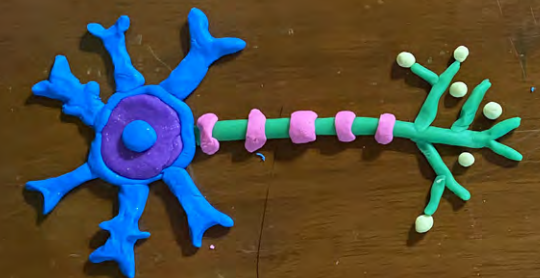
Sample motor neuron created from playdough. Image credit: Center for the Integrative Study of Animal Behavior/Indiana University
Once students have completed the activity ask them to reflect on the following questions:
- What do you think a motor neuron does?
- Why would this shape and structure help it carry out its function?
Motor neurons connect the central nervous system to muscles throughout the body. The long axon can carry signals across long distances.
Lesson 2: Action Potentials
Objective: Students will be able to describe how electrical signals are carried from one end of neuron to the other both through myelinated axons and non-myelinated axons.
ENGAGE/HOOK: Electricity in our Bodies (10 min)
Introduce students to the idea of an action potential by showing them the first few minutes of this Crash Course video about how the nervous system works (stop at 3:18).
Following the video, have students independently reflect on the following questions:
- How is our body like a “sack of batteries”?
- Explain how the flow of electricity is similar to what happens in a neuron.
EXPLORE: Action Potential Simulation (30 min)
Have students work through this online interactive action potential simulation using the Action Potential student worksheet independently or in pairs.
Refer to the Action Potential teacher guide for answers.
Ask these discussion questions after students have completed the simulation.
- How is the axon like a battery? How is energy stored?
- recall that the charges are separated across the cell membrane
- How does the cell keep the charged ions separated at the right concentrations?
- ion channels (both gated and leak channels) maintain the correct concentration
- Why does the membrane potential need to recharge before another action potential can take place?
- to ensure that the action potential can fire at full strength and the signal is not lost or weakened
EXPLAIN: Action Potentials and Myelination
The following information is teacher-facing and can be utilized to teach students new information in whatever format works best for you and your students.
Key Points:
- Most neurons have a negative charge inside the cell compared to outside the cell which creates an electrical potential across the cell membrane of the axon (the membrane potential).
- An action potential is activated when an incoming stimulus reaches the threshold for depolarization (a change in charge).
- An action potential is an all-or-nothing event—the size of the action potential is always the same but a larger stimulus may produce a higher frequency of action potentials.
- Because of the electrical nature of an action potential, a myelin coating around an axon can provide insulation to help the signal travel faster.
Action Potentials
Action potentials are the way that a signal in the nervous system travels from the cell body to the very end of the axon, as shown in the diagram below. An action potential is an extremely fast way to transmit signals, and is electrical in nature. The two important “forces” that lead to an action potential are electrical (differences in charge across the membrane, between the inside and outside of the neuron) and chemical (differences in ion concentration across the membrane, between the inside and outside of the neuron).
At rest, most neurons have a negative charge inside the cell compared to outside the cell which creates an electrical potential. If a stimulus arriving at the neuron is large enough to raise the membrane potential up to a certain threshold, a process will begin that quickly depolarizes (makes more positive) and then repolarizes (returns to rest) the cell. This quick and temporary change in charge is the action potential. Action potentials travel down the axon as a wave of sodium and potassium ions, depolarizing and repolarizing sections of the membrane as they go. You can refer back to the simulation to review these stages of the action potential.
Action potentials are “all-or-none” meaning either the initial stimulus is large enough to initiate an action potential or the cell’s charge quickly returns to rest and no action potential is triggered. The magnitude and duration of a given cell’s action potential do not differ based on the strength of the incoming stimulus, though the strength of the stimuli does influence the number and frequency of action potentials produced.
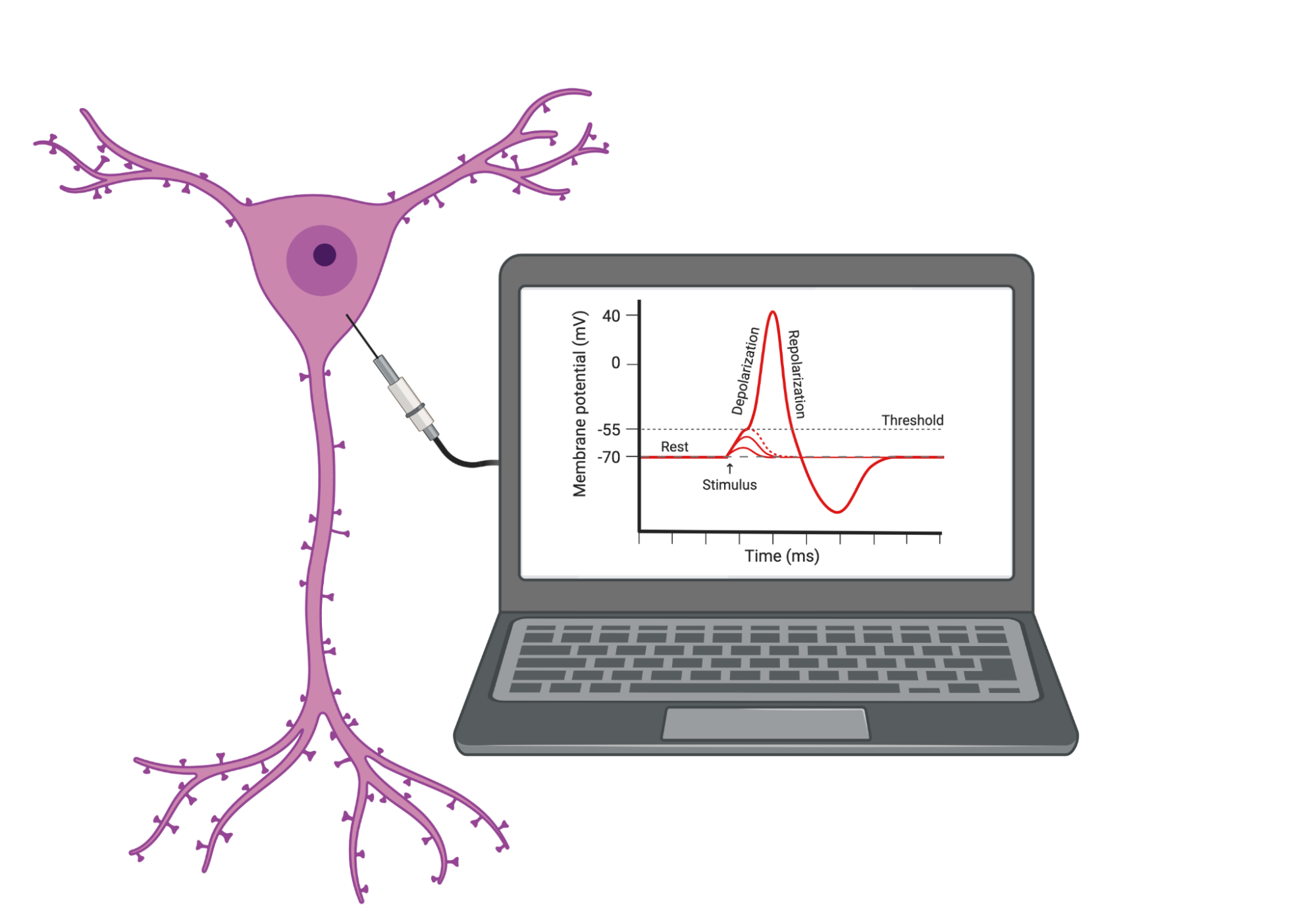
The action potential is a change in charge that can be recorded using electrodes inserted into the neuron itself and imaged on an oscilloscope or computer. Image credit: BioRender.com
Myelination
To make the action potential process even faster, many axons are coated in myelin, a fatty substance produced by specialized glial cells. Myelin essentially insulates the axon so that signals can travel farther and faster, much like how copper wires are often wrapped in rubber insulation. In addition to increasing the speed of neural transmission, myelin also helps to protect the axon from damage and helps to prevent the leakage of ions across the membrane, which can disrupt the electrical signal. Although not all neurons have myelinated axons, overall, myelination is critical for proper neural functioning and is essential for processes such as motor coordination, sensory perception, and cognitive processing. Its importance is demonstrated by the often severe symptoms of multiple sclerosis, a disease in which your immune system starts to attack myelin and disrupts the transmission of signals to and from your brain. “White matter” describes the areas of the brain where many of these myelinated axons reside. Because fat is white, this is how white matter gets its color! More about white and gray matter is covered in Lesson 5 of this unit.
Additional Resources
- This article has a simple description of the action potential, with a few quiz questions at the end (Biology Workbook for Dummies).
- This video explains how charge moves during an action potential. (Harvard University Extension School)
- This video explains how traumatic brain injuries affect neurons, using football concussions as an example. More about sports and traumatic brain injuries is covered in Unit 4, Lesson 4. (The Franklin Institute)
- This website includes audio recordings of what firing neurons “sound” like if the electrical activity of the action potential is run through an oscilloscope that matches sound waves to the electrical wavelengths. Look for the two recordings of trigeminal ganglion cells and listen for the differences between them.
ELABORATE: Modeling Myelination (10 min)
Explain the background information below to students to frame the following activity:
In this lesson we investigated action potentials—the way that a signal in the nervous system travels from the cell body to the very end of the axon. To end the lesson we will model two ways that signals can travel in the nervous system: with non-myelinated axons and with myelinated axons.
- Have ten students link hands (representing a non-myelinated axon)
- Have five other students link hands but stretch to the same length (representing a myelinated axon)
- When you say “go”, the first student in each line, squeezes the hand of the next student, and so-on down the line.
- Try this a few times and see who wins each time.
It should take the longer line about twice as long to transmit the hand-squeeze signal to the end which is a good model of how myelin helps a signal “skip” along the length of the axon. - Ask students to reflect on the demonstration with the following questions:
- Which group passed the “signal” fastest? Why?
- Which group represented a non-myelinated axon and which group represented a myelinated axon? How do you know?
The group of 5 students represent axons that are coated in special fatty membranes called myelin that allows signals to travel faster. See this activity guide for acting out saltatory conduction (page 12) for more details and suggestions of how to measure the time of transmission to extend the activity to include working with quantitative data.
Lesson 3: Synaptic Transmission
Objective: Students will be able to explain how chemical neurotransmitters help to carry signals across the synapse (space) between neurons and how different neurotransmitters have different effects on the brain.
ENGAGE/HOOK: Neurotransmission (15 min)
Introduce the lesson by explaining the background information below and guiding them through the following activity:
Remind students that the last lesson was about how signals travel from one end of a neuron to the other—action potentials are electrical signals within a single neuron. Explain that this lesson will focus on how a message is transferred from one neuron to the next.
- Ask students to draw and label a model of how they THINK neurons communicate with each other. Remind them of the structures of the neurons they already know and that there are spaces (synapses) between the individual neurons.
- Ask students to share out their models and compare them to their classmates’.
- Once students have thought of their own models, show them this video about how neurons communicate from BrainFacts.org and ask them to reflect on the following questions:
- This video explains how messages travel in neurons and from one neuron to the next. Within one neuron how does the message travel?
- What happens to the message at the synapse, the space between two neurons?
- What two things can happen as the chemical message from one neuron crosses the synapse and interacts with the next neuron?
EXPLORE: Common Neurotransmitters (20 min)
Guide students through the following activity which will help establish a baseline of student interest and connect their knowledge to further exploration. Students will research a familiar neurotransmitter to identify its context in pop culture (using popular media headlines, social media, music, movies, etc.) and research its functions in the brain.
- Explain this background info to students:
The brain uses a number of different chemical neurotransmitters to carry the signal across the space between neurons. These neurotransmitters work in different combinations and with different effects on the neural signals. Some of these neurotransmitters may be familiar to you (note that some of these chemicals also function as hormones in other parts of the body, so you may suggest that they narrow their search by adding “neurotransmitter,” “brain,” or other brain-specific terms), especially in cases where they have been found to be closely associated with emotions or other specific brain functions of popular curiosity. - Ask students to choose one of the neurotransmitters in the table below to research.
- Ask students to answer the following questions about their neurotransmitter:
- What neurotransmitter did you choose and what is it associated with?
- What perceptions might non-scientists have about this neurotransmitter?
- Where do you find popular references to its function? Is it associated with any over-the-counter supplements or common medications?
- Where in the brain is this neurotransmitter produced? What are its typical functions in the brain?
- What are some symptoms that might occur if this neurotransmitter is not functioning properly?
Neurotransmitter | Commonly associated with… |
Serotonin | Boosting your mood |
Adrenaline/Epinephrine | Thrill seeking, stress |
Dopamine | Happiness |
Oxytocin | Love |
GABA | Calm and relaxation |
Note to teachers: Some students may have heard of some of these neurotransmitters before and have a perception that one neurotransmitter controls one emotion or function. For example, they may have heard that dopamine makes you “feel good” or that serotonin makes you feel relaxed after a good meal. This is true but too simplistic - each neurotransmitter controls many different functions in many different regions of the brain!
EXPLAIN: Synaptic Transmission
The following information is teacher-facing and can be utilized to teach students new information in whatever format works best for you and your students.
Key Points:
- Synaptic transmission is the process by which a signal is communicated from one neuron to the next, using chemicals called neurotransmitters.
- There are many different neurotransmitters that work in different combinations, contributing to the complex signaling in the brain.
- Neurotransmitters can trigger fast responses (via ions) or slower responses (via second messenger molecules) in the neuron receiving the signal.
When the action potential reaches the axon terminal/synapse, a chemical process called synaptic transmission, or neurotransmission, begins. Neurotransmitters carry the signal from the axon (the presynaptic cell) to the dendrite of another neuron (the postsynaptic cell). There are over 100 identified kinds of neurotransmitters, though each neuron only uses a small number of these. Knowing about different neurotransmitters is the key to understanding how drugs influence behavior (see more information about drugs and their mechanisms of action in Unit 5, Lesson 2).
The steps of synaptic transmission can be seen in the figure below. Vesicles filled with neurotransmitters (NTs) that were produced in the cell body (1) wait in the terminal. When triggered by an action potential arriving at the terminal (2), the vesicles bind to the membrane (3) and release the NTs into the “synaptic cleft,” or the space between the pre and postsynaptic neurons (4). These NTs bind to receptors on the postsynaptic cell, which then trigger an effect on that cell (5). These effects can either be localized and fast (via allowing ions to flow into or out of the postsynaptic dendrite) or slower but more widespread (via second messenger molecules spreading within the postsynaptic cell, as explored in the Neurotransmission Game below). Many neurons use a transporter molecule to recycle the NTs (6), bringing them back into the presynaptic cell to be repacked into vesicles and reused.
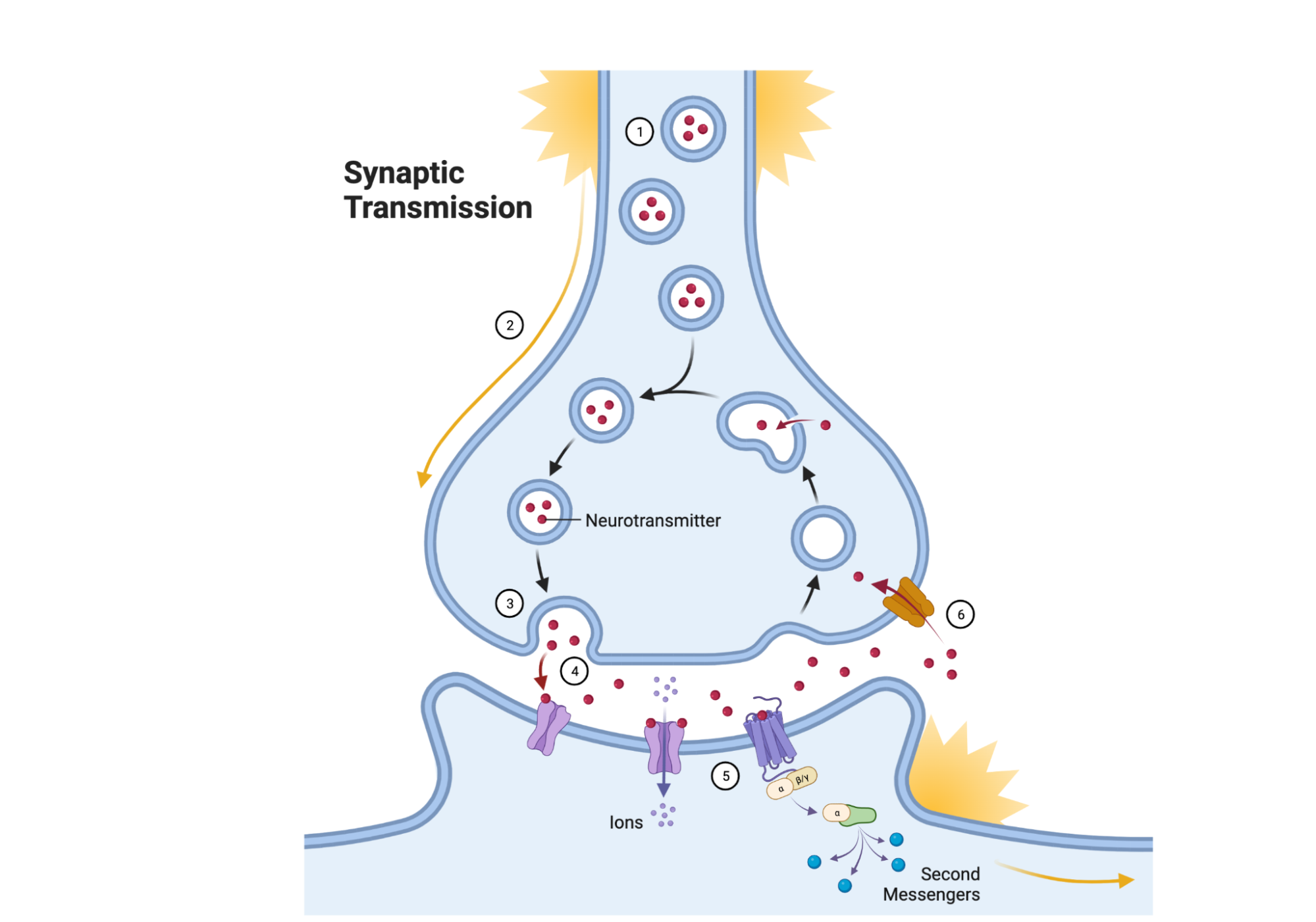
Neurotransmission is the cycle through which chemical neurotransmitter molecules are released in order to bring information to the postsynaptic cell, and are then often recycled or broken down. Image credit: BioRender.com
Additional Resources
- This video explains how synaptic transmission works through narrated hand-drawn diagrams. (Neuroscientifically Challenged YouTube channel)
- This video has some interesting animations demonstrating the function of neurotransmitters. (Nancy LeNezet, IB Psychology teacher)
- This fact sheet is a reference for the parts of a neuron. There is also fill-in-the-blank activity on pages 1-4 that could be used to solidify the terminology. (National Institute on Drug Abuse)
- This website is a thorough guide to neurotransmitters and how they work. (Cleveland Clinic)
ELABORATE: Neurotransmission Game (30 min)
Guide students in this large group activity for modeling neurotransmission that demonstrates the different components involved.
- Break students into teams of 7-8
- Assign students in each team to the following roles:
- Presynaptic action potential (1-2 per team)
- Neurotransmitter vesicle (1 per team)
- Receptors (2 per team)
- Second messengers (2 per team)
- Transporters (1 per team)
- Each team forms a line.
- Have the “presynaptic action potential” students stand a short distance away from the rest of the line.
- Say “go” to start the game. The presynaptic action potential students should run down and tag the neurotransmitter vesicle, then return to their starting position.
- When tagged by the action potentials, the vesicles throw a “neurotransmitter” (ping pong ball) across a gap to the receptors who catch it in their hands, a plastic cup, bowl, or large spoon (depending on how difficult you want to make the game).
- Once they’ve caught the ball, the receptor tags the second messenger students (who are standing right by them). The second messengers run over to the whiteboard and tally a point for their team to show depolarization. They then run back and tag the receptor.
- After the receptor is tagged, they throw the ball to the transporter, who then gives the ball back to the vesicle.
- Once the vesicle has the ball, the presynaptic action potential can restart the process.
Teacher notes:
If a receptor drops a ball, it is out of play. The transporter must kick the ball and return it to the vesicle to restart. The receptor cannot pick up any dropped balls, as receptors are bound to the cell membrane.
Once a team has tallied 10 points on the board, they have fired an action potential in the postsynaptic neuron and they win.
To see this game in action, watch two teams of students model the procedure.
EXTEND: Reading Research
The following activity can be used to extend the lesson through a jigsaw or as an at-home assignment.
Science in the Classroom (SitC) is a collection of annotated research papers and accompanying teaching materials designed to help students understand the structure and workings of professional scientific research.
This annotated paper, “Overcrowding in Neuronal Synapses” studied how making a 3D computer model of a synapse can tell us more about how neurons use synapses to communicate with each other.
- Assign small sections of the article to student groups to read and discuss during class
- Have each group present or use a jigsaw method to teach the entire class what is in their part of the article.
- See the Teacher Resource guide that accompanies the article.
Section 2: Brain Anatomy
Lesson 4: Lesion Studies
Objective: Students will be able to explain how case studies of brain lesion patients revealed the roles of certain brain regions.
ENGAGE/HOOK: Imagining Lesion Effects (20 min)
Note to teachers: This lesson will utilize this document describing real-life case studies of people with brain lesions several times. Please read and familiarize yourself with the case studies in advance.
Explain the background information below to students to frame the following activity:
Today’s lesson will be about brain lesions, or areas of the brain that have shown damage, and how studying them have revealed insights into brain function and structure. As you'll see today and in other examples throughout the course, these case studies often involve unfortunate circumstances in people's lives, including trauma and injury. It's important to talk about these cases because they have been fundamental to our understanding of the brain due to limitations in technology (noninvasive scans weren’t invented until the mid 20th century) and the ethical challenges of experimenting on human brains. These medical cases of brain injuries and traumas can be studied as natural experiments that provide insights into the brain without causing intentional harm.
To begin thinking about lesions we will imagine, act, and analyze scenarios based on a few real-life case studies.
- Assign groups of students one case study from this document.
- It may be helpful to use this Reading for the GIST template to support students as they unpack the case studies.
- Assign one student the role of the lesion patient. The other students can play other characters interacting with the patient.
- Students must create a scenario that demonstrates the patient’s deficit.
- While one group performs the scene, the other students watch and compile a list of symptoms and/or deficits that they notice.
- Once all of the groups have performed their scenes, lead a discussion using the symptom lists, to talk about the specific region and function of that part of the brain.
EXPLORE: Lesion Map (30 min)
Guide students in the following activity to help them dive deeper into the same case studies they acted out in the Engage section. Student groups will need one copy of the real-life case studies document and one copy of the student worksheet.
- Re-assign student groups to the same case study from the real-life case studies document that they acted out in the Engage.
- Ask student groups to re-read their case study and fill out the questions on the first page of the student worksheet. The questions are also listed below:
- What part of the brain was damaged?
- How did the patient’s behavior or abilities change as a result?
- What function do you think this part of the brain is responsible for?
- Then have student groups create a heuristic diagram (on the second page of the student worksheet) of brain areas and functions based on their conclusions for their case study.
- After students have completed the worksheet, have each student group present their cases and heuristic diagrams to the class. Compare what they created to the teacher guide.
EXPLAIN: Why Study Brain Lesions?
The following information is teacher-facing and can be utilized to teach students new information in whatever format works best for you and your students.
Key Points:
- Changes in the brain cause various changes in cognition and/or behavior.
- Different cognitive functions can be localized to different parts of the brain.
- Neuroscientists can learn about the brain by examining patients with damage due to stroke or injury: by studying how behavior changes when a region of the brain is damaged, we can infer how that brain region normally works.
Mapping Brain Functions
Is the brain a uniform mass of tissue, or are different parts responsible for different mental functions? Patients with brain lesions helped answer this question. Historically, when a patient would show up to the clinic after a stroke or acute head injury, doctors would record the specific behavioral changes or deficits and then look to see which part of the brain was damaged (back then, the patient usually had to die before the doctor could perform an autopsy to discover the site of damage). Because damage to a specific area tended to cause the same behavioral changes across patients, doctors were able to begin mapping mental functions to specific regions of the brain. This method is still a common way to study the relationship between brain regions and function, but now with brain imaging (see Unit 3, Lesson 1), doctors can locate the site of damage while the patient is still alive.
Experimenting with Lesions
Lesions can be experimentally generated in animals to investigate the neural mechanisms underlying various behaviors and cognitive functions, contributing to our understanding of human brain function and the development of treatments for neurological disorders. Similarly, observing lesions in patients with neurological disorders or damage (see examples below) has been used to develop new treatments that target specific regions of the brain. Finally, when a brain region is damaged, other regions may compensate for its functions. This phenomenon is known as brain plasticity. By studying the changes that occur after a lesion, researchers can gain insights into the brain's capacity for reorganization and recovery.
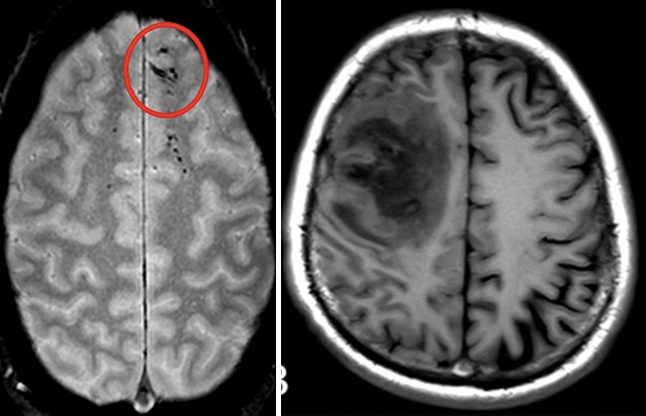
Examples of lesions include a brain microbleed (left) and a lesion in the left frontal lobe (right). Image credits: National Institute of Neurological Disorders and Stroke/NIH (left); adapted from "CT and MRI scan of the brain with melioidosis" by Wei-yuan Huang, Gang Wu, Feng Chen, Meng-meng Li and Jian-jun Li is licensed under CC BY 4.0 (right)
Additional Resources
- Mapping Brain Functions
- This article provides a good perspective on why teaching with case studies is an effective approach to student learning in neuroscience. (Journal for Undergraduate Neuroscience Education)
- Read more about some of the real-life case studies and the importance of lesion methods in neuroscience. (Frontiers for Young Minds)
- Experimenting with Lesions
- This remarkable case study of a boy who can see despite lacking much of his visual cortex demonstrates the incredible neuroplasticity of a young child’s brain. (Science Alert)
ELABORATE: First-Person Narrative (20 min)
Ask students to pick one of the 8 disorders in the real-life case studies document and write a first-person narrative about what life might be like living with the lesion, described from the perspective of the patient. Depending on the length of the assignment, the narrative could also include how the patient acquired the disorder and how their symptoms may have changed over time.
Lesson 5: Parts and Regions of the Brain
Objective: Students will be able to identify major brain regions and describe their primary functions through the use of multiple interactive brain-study tools.
ENGAGE/HOOK: Virtual Brain Dissection (15 min)
To dive deeper into the parts of the brain, allow students to explore this interactive 3-D brain model from BrainFacts.org which has a rotatable (and see-through) brain. This interactive is very helpful when trying to understand where the different parts of the brain are in a 3-dimensional space. It has a large number of labeled parts and descriptions of function.
Brain Model Directions:
- Holding and moving the cursor on the brain will rotate it.
- Selecting areas of the brain will isolate that area and give you a description.
- The drop down menu on the top left allows you to select a brain area to isolate and learn about.
- The slider at bottom allows you to “disassemble” the brain to isolate a brain area.
As students explore ask them to answer these guiding questions:
- Click and learn about the right/left cerebral cortex and then the areas of the brain stem. Compare their functions. How are the roles of the cortex and brain stem different?
- What areas of the brain are important for keeping your body alive, like regulating heartbeat and breathing?
- Can you find the area of the brain that controls speech? Certain behaviors? Decision making?
EXPLORE: Shower Cap Mind Map (30 min)
In this activity, students will label parts of the brain on the head of one of their classmates. This article from Advances in Physiology Education describes the Shower Cap Mind Map activity and has examples of the brain regions students should label.
- Split students into teams of 3 and assign one student to wear a shower cap.
- The rest of the team uses sticky notes to label parts of the brain on the shower cap.
- Labels can be brain lobes, hemispheres, sensory processing regions (e.g. primary auditory or visual cortex), etc.
- Give teams a limited amount of time to correctly place the labels.
- As an alternative, there is a premade Brain Hemisphere Hat template designed by Ellen McHenry. This works best when scaled to print on 11” x 14” paper to fit on a student’s head.
EXPLAIN: Brain Regions
The following information is teacher-facing and can be utilized to teach students new information in whatever format works best for you and your students.
Key Points:
- The anatomy of the brain can be characterized in multiple ways that provide orientation to overall structure and functional locations.
- Individual regions of the brain are typically defined by their primary roles, but many regions work together to carry out complex functions.
Overall Organization of the Brain
The brain is an extremely complicated part of the human body, but learning a few orienting terms makes it easy to navigate, and learning some of the major parts and functions goes a long way in understanding how mental processes arise.
The brain is divided into two hemispheres: left and right. Each hemisphere has distinct functions, but they are always working together. For sensory input and voluntary motor functions, the hemispheres are symmetrical—the right hemisphere coordinates the left side of the body while the left hemisphere coordinates the right side of the body.
Another way of looking at the brain is from the inside out, looking at cross sections cut through the brain to see the parts inside. Brain tissue is usually either gray matter or white matter. Gray matter is made up of cell bodies and dendrites of neurons, as well as glia. White matter is mostly the myelinated axons of neurons.
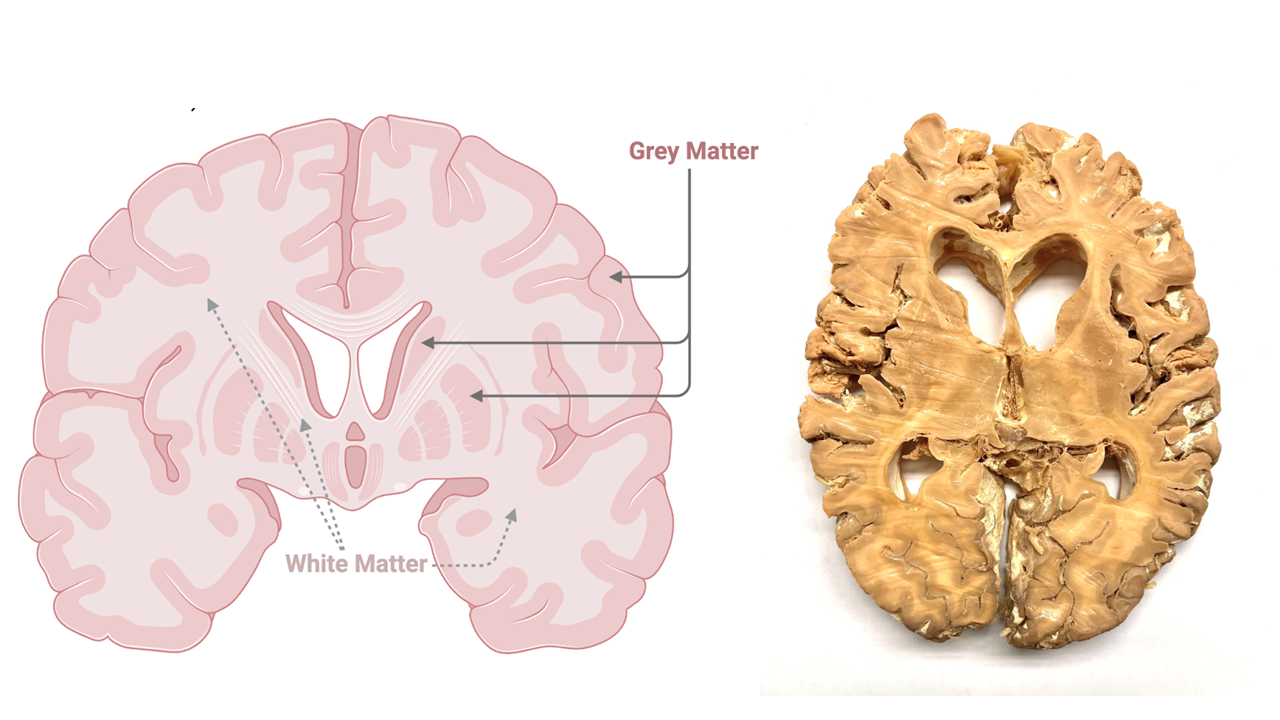
A cross section of a brain, presented as a diagram on the left and a plastinated human specimen on the right, shows the pale white-ish parts inside the brain, called white matter, which are made up of myelinated axons. The gray matter can be found in small clusters deep inside the brain and around the outer edge (forming the cerebral cortex). Diagram credit: BioRender.com
The cerebral cortex is the wrinkly layer of gray matter found on the outside—this is where most of our thought processing happens. The brain stem is at the core and bottom of the brain, controlling automatic functions of the body and connects to the spinal cord. The cerebellum is the small section at the back, sitting under the cerebral cortex, and controls coordination and balance.
The brain is also divided into four main lobes: frontal, parietal, temporal, and occipital, that help orient us to the anatomy of the brain. Many brain functions involve interactions between multiple lobes, but there are key functions that can be roughly geographically assigned to each lobe:
- The frontal lobe is associated with decision-making, planning, judgment, and motor control.
- The parietal lobe processes sensory and spatial information and integrates information coming from different senses.
- The temporal lobe includes regions responsible for sound processing, language comprehension, and memory formation.
- The occipital lobe is primarily responsible for processing visual information, including color and motion detection.
Image
The main part of the brain is divided up into 4 main sections or lobes, based on physical landmarks and the functions or roles of each brain area. The cerebellum and the brainstem are not considered to be part of any lobe. Image credit: BioRender.com
Notable Brain Areas
Within the four main lobes, there are regions and structures of the brain that have been identified for their association with specific functions (refer back to the 3-D virtual brain map for exact locations). A few notable ones to mention are:
- Corpus callosum - a region of white matter that connects the left and right hemispheres.
- Amygdala - this region is involved with emotional response, including fear and motivation.
- Hippocampus - this region plays an important role in forming long-term memories.
- Thalamus - this is the “air traffic control” center of your brain, relaying information from all your senses (except smell) and sending it to the frontal lobe for interpretation.
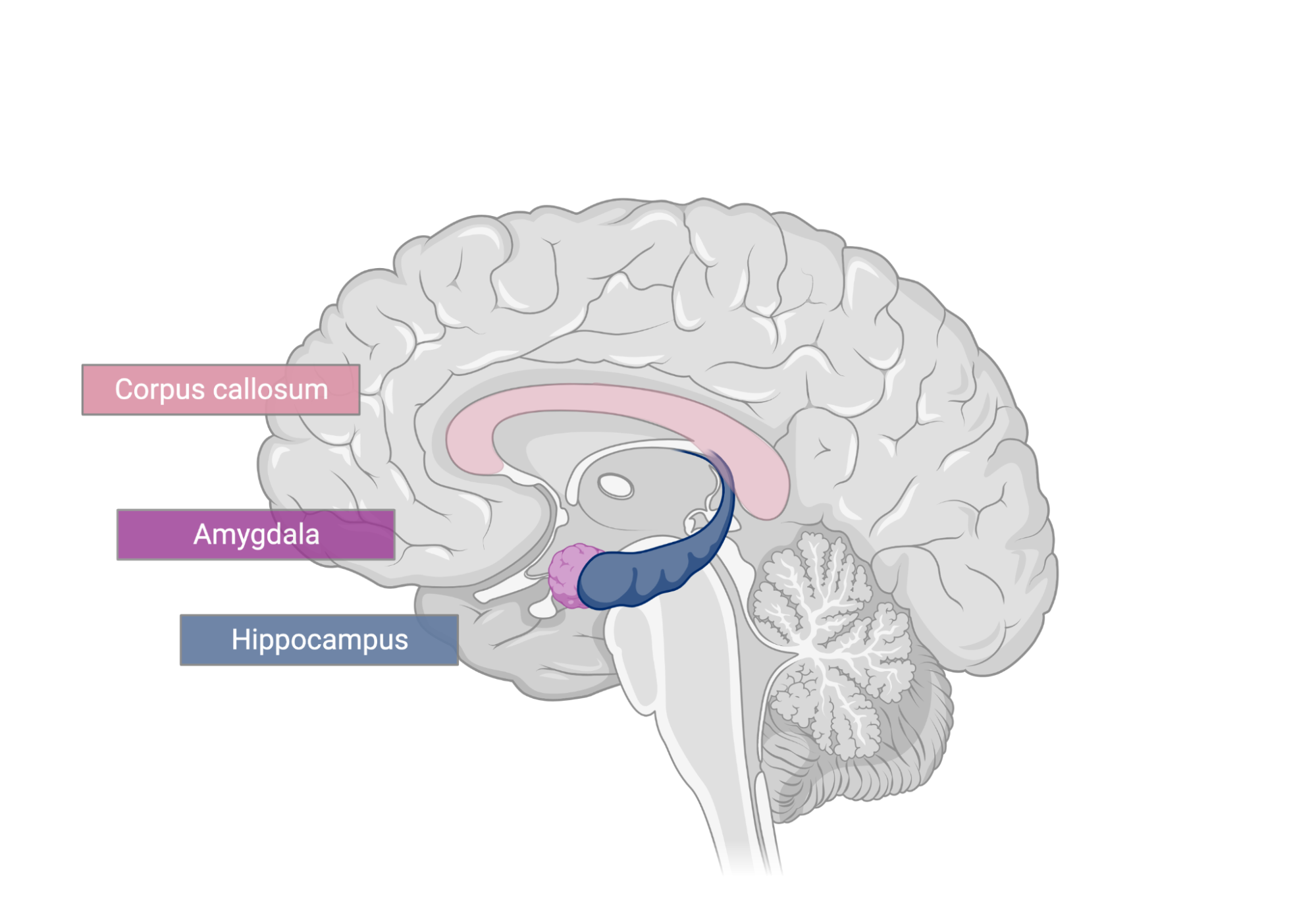
The corpus callosum, amygdala, and hippocampus reside deep inside the brain. Image credit: BioRender.com
Additional Resources
There are many resources online for learning about brain anatomy and function, though note that many will go into more detail than necessary for a basic overview.
- This website for teachers goes over basic brain anatomy and orientation terms, and has links to other similar lesson plans and study guides. (BrightHub Education)
- This video discusses the reality of the right and left hemispheres of the brain and how they function. (TED-Ed)
- This tutorial has good visual descriptions of the various orientation terms (superior, inferior, etc.) and the reference planes (coronal, horizontal, sagittal). It also has good visual and text descriptions of cortical regions. Introducing terminology for orientation and reference planes is optional; while it can be useful to connect to an anatomy lesson, for instance, it is not required in the context of this curriculum. (GetBodySmart)
- This video includes some segments that help illustrate a 3-dimensional understanding of brain anatomy. (AnatomyZone)
ELABORATE: Turn Off Your Perception (10 min)
- Have students explore this interactive simulation to deactivate different brain regions.
- Ask students to reflect with these questions:
- Which regions control which functions?
- What happens when multiple brain regions are deactivated? What do you think this means about how different brain regions work together?
EXTEND: Poems and Dissections
Below are two possible extensions you could add to this lesson OR make this a two-part lesson including the activities below. The dissection activity would likely need its own separate lesson.
Sheep Brain Dissection
Depending on the size of the class, individuals or teams dissect a sheep brain, which is anatomically similar to a human brain and less than $15 per brain.
- Sheep brains can be purchased from Carolina Biological Supply Company.
- In some areas local meat processing plants may also be an option for procuring brains.
- This website has great sheep brain dissection resources, including a teacher curricula, student worksheet, and videos. (University of Notre Dame BrainU)
- This website includes student worksheets for labeling parts of the sheep brain. (Home Science Tools)
- This video demonstrates a sheep brain dissection. (Glen Oaks Community College)
- This two-part video demonstrates the dissection in more detail (Sheep Brain Dissection Part 1, Sheep Brain Dissection Part 2). (BioBeware)
- As an alternative to dissection, there are gelatin brain molds that can be used to label lobes and practice dissections, slice orientations, and anatomical direction terms (e.g. take a coronal slice from the most posterior portion of the occipital lobe).
Lobe Poems
Individuals or teams create short poems to describe a particular lobe of the brain. Those who didn’t write the poem guess which lobe the poem is describing.
Example poem:
This part of the brain helps you move,
Without it you couldn’t bust a groove
Coordinating movement for a cool guy swagger,
Without it you’d look drunk and stagger.
Can you guess what part of the brain this is? Answer: cerebellum.
Lesson 6: Comparative Neuroanatomy
Objective: Students will be able to compare the size of human brains to other species' brains and give examples of how brains have evolved for the needs of different species.
ENGAGE/HOOK: How We Sense Our Environment (15 min)
Introduce the lesson by explaining the background information below and guiding them through the following activity:
This final lesson will focus on the comparison of neuroanatomy between humans and other animals. Because all of our senses are ultimately processed in the brain, the differences in how animals experience the world relates to differences in the brain. For example, the part of our brain that processes visual input is very large because this is our most well developed sense. The following activity will allow you to explore sensory differences between animals.
Have students complete the Sensing Our Environment student worksheet (teachers may refer to the Sensing Our Environment teacher guide) while listening to the Radiolab podcast “Colors”(skip to 8:30-17:30 for a comparison of different species and their color vision).
EXPLORE: Modeling Animal Brains (30 min)
Guide students through the following hands-on activity to compare animal brains to human brains:
- Have students pick their favorite animal.
- Ask students to look up images of that animal’s brain online in comparison to a human brain. Search “brain size of __ compared to humans.” Two example images are shared below.
- Ask students to shape that animal’s brain out of Play-Doh or clay.
- Student models should be to scale (since size is an important part of brain differences), but the larger brains may be scaled down.
- Once students complete their brains have them discuss:
- How is your animal’s brain different from and similar to a human brain?
- How is your animal’s brain different from and similar to the other animal brains your classmates made?
(This activity could also be used as another way to teach students the basics of human brain anatomy. See this Clay Brains activity guide from the University of North Carolina for an example.)
Sample image showing brains from several different mammals and the number of neurons found in their cerebral cortices. (Quanta Magazine)
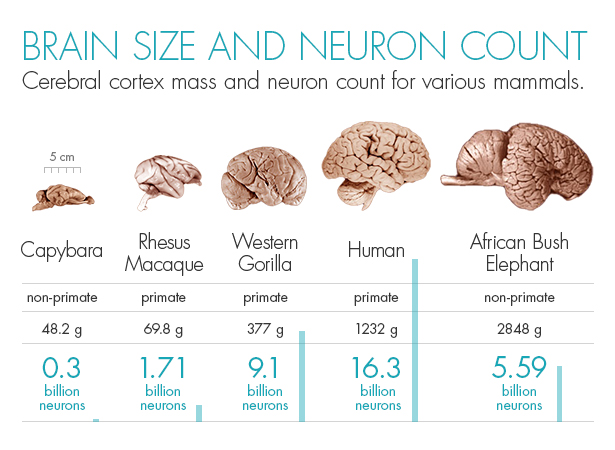
Although elephants are physically much larger than primates, they do not have as high a proportion of their brain dedicated to the cerebral cortex as many primates. Image credit: Olena Shmahalo/Quanta Magazine
Another example image comparing the overall size of several different mammalian brains. (McGill University)
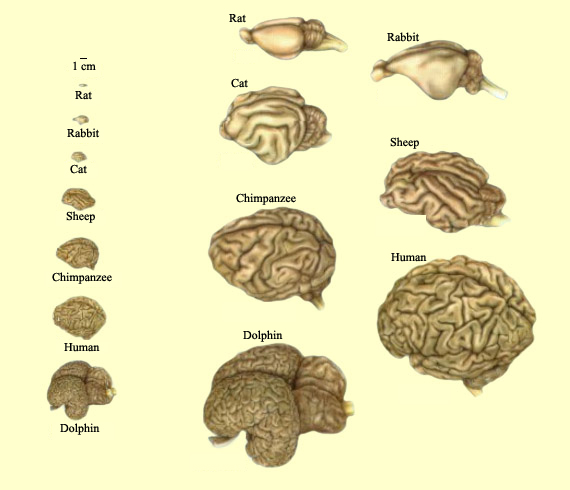
The more cerebral cortex an animal’s brain has, the more wrinkly the brain appears, as the sheet of neurons that forms the cerebral cortex must be crumpled up to fit inside the limits of the skull. Image credit: McGill University
EXPLAIN: Comparative Neuroanatomy
The following information is teacher-facing and can be utilized to teach students new information in whatever format works best for you and your students.
Key points:
- Comparing species brains can highlight differences and can be important in medical research
- The overall size of a brain is not the most important factor in determining intelligence (e.g. humans have nowhere near the biggest brain in terms of overall size).
- The brain-to-body mass ratio, as well as the amount of “wrinkles” on a brain (surface area to volume ratio), is a rough measure of the intelligence of an animal—but not without flaws.
- Frontal lobe areas relative to the rest of the brain are biggest in humans.
Comparing Similarities and Differences
Brains are similar in many ways between species when it comes to general anatomical structure and function. These similarities allow us to learn more about our own brains and behavior by studying other animals. At the same time, there are also many differences between species, particularly when it comes to size relative to body mass and the ratio of surface area to volume (i.e., a more “wrinkly” brain structure means there is more brain tissue folded into the cranial cavity). Comparing species is also important for medical research, as it can provide insights into the causes and treatments of neurological disorders. For example, by studying the nervous systems of different animals, researchers can identify potential targets for new drugs and therapies.
Evolution, Size, and Complexity
Comparative neuroanatomy helps us understand the evolutionary relationships between different species and determine how changes in the nervous system have contributed to the diversification of life on Earth. One area of interest is the size and complexity of the brain. For example, the brain of a human is much larger and more complex than that of a fish, but the fish brain is still capable of performing a range of complex behaviors. Also, the relative size of brain regions within a species tells us what functions are particularly important to that species. For example, compared to other species, humans have larger frontal lobes relative to the rest of their brain. Frontal lobe regions are responsible for mental processes such as reasoning, decision-making, planning—things that humans are very good at doing!
Additional Resources
- Comparing Similarities and Differences
- One of the best resources for images and descriptions of a variety of animal brains is the Comparative Mammalian Brain Collections. (University of Wisconsin and Michigan State Comparative Mammalian Brain Collections, National Museum of Health and Medicine)
- This overview of the brain-to-body mass ratio summarizes our understanding of the physiological relationship as well as caveats. (Wikipedia)
- Evolution, Size, and Complexity
- This article lays out some of the ongoing discussion about brain size, organization, and intelligence differences between species. (Scientific American)
- This article provides a good overview of how animal models are used in neuroscience research. (European Animal Research Association)
- An option for extending the discussion of comparative neuroanatomy is to think about intelligence: Are humans smarter than other animals? What does “smarter” mean, anyway?
- There is much disagreement about how intelligent other animals are. For example, this article describes the conflicting evidence about the intelligence of dolphins. (Discover Magazine)
- It is important to remember that different species have developed sensory systems appropriate for their environment and needs. While humans are more intelligent than other species, they are not better at everything. For example, when it comes to sensory processing…
- Dogs have a better sense of smell than humans do.
- Butterflies can see more colors than humans do, including UV light.
- Bats can echolocate, while humans can’t.
- Read about more examples of animal sensory processing abilities. (Discover Magazine)
ELABORATE: Animal Models in Neuroscience (30 min)
Explain the background information below to students to frame the following activity:
The similarities between the nervous systems of different species allow researchers to experiment with animals to learn more about humans.
- Have students read this article summarizing strengths and weaknesses of studying animal models. (Dana Foundation)
- Ask students to select a species (options and things to consider listed below) and investigate the use of that species as an experimental model for neuroscience research.
Species used in neuroscience research: Factors to consider: - Round worms (Caenorhabditis elegans)
- Fruit flies (Drosophila melanogaster)
- Zebrafish (Danio rerio)
- House mice (Mus musculus)
- Brown rats (Rattus norvegicus)
- Rhesus monkeys (Macaca mulatta)
- Or other less common species, such as desert locusts, frogs, fruit bats, ferrets, zebra finches, lobsters, chimpanzees, etc.
- Similarity to human brain anatomy, function, or behavior
- Existing knowledge of species’ genetics or ability for genetic manipulation
- Logistics of maintenance
- Generation time and
- Moral and ethical considerations
- Students should answer the following questions about their chosen species:
- What animal did you choose?
- What are some overall benefits and limitations to using this species as an experimental model?
- What is one question that researchers have investigated using that species? Why was that species selected?
- What insights into the human brain were discovered by studying this animal?
For more information about the Neuroscience & Society Curriculum, please contact neuroscience@fi.edu.
Neuroscience & Society Curriculum
Launch Lesson • Unit 1: Neurons and Anatomy • Unit 2: Education and Development • Unit 3: Current Methods in Neuroscience • Unit 4: Mental Health and Mental Health Conditions • Unit 5: Drugs and Addiction • Unit 6: Law and Criminology • Unit 7: Future Technologies

This project was supported by funding from the National Institutes of Health Blueprint for Neuroscience Research under grant #R25DA033023 and additional funding from the Dana Foundation. Its content is solely the responsibility of the authors and does not necessarily represent the official views of NIH or the Dana Foundation.