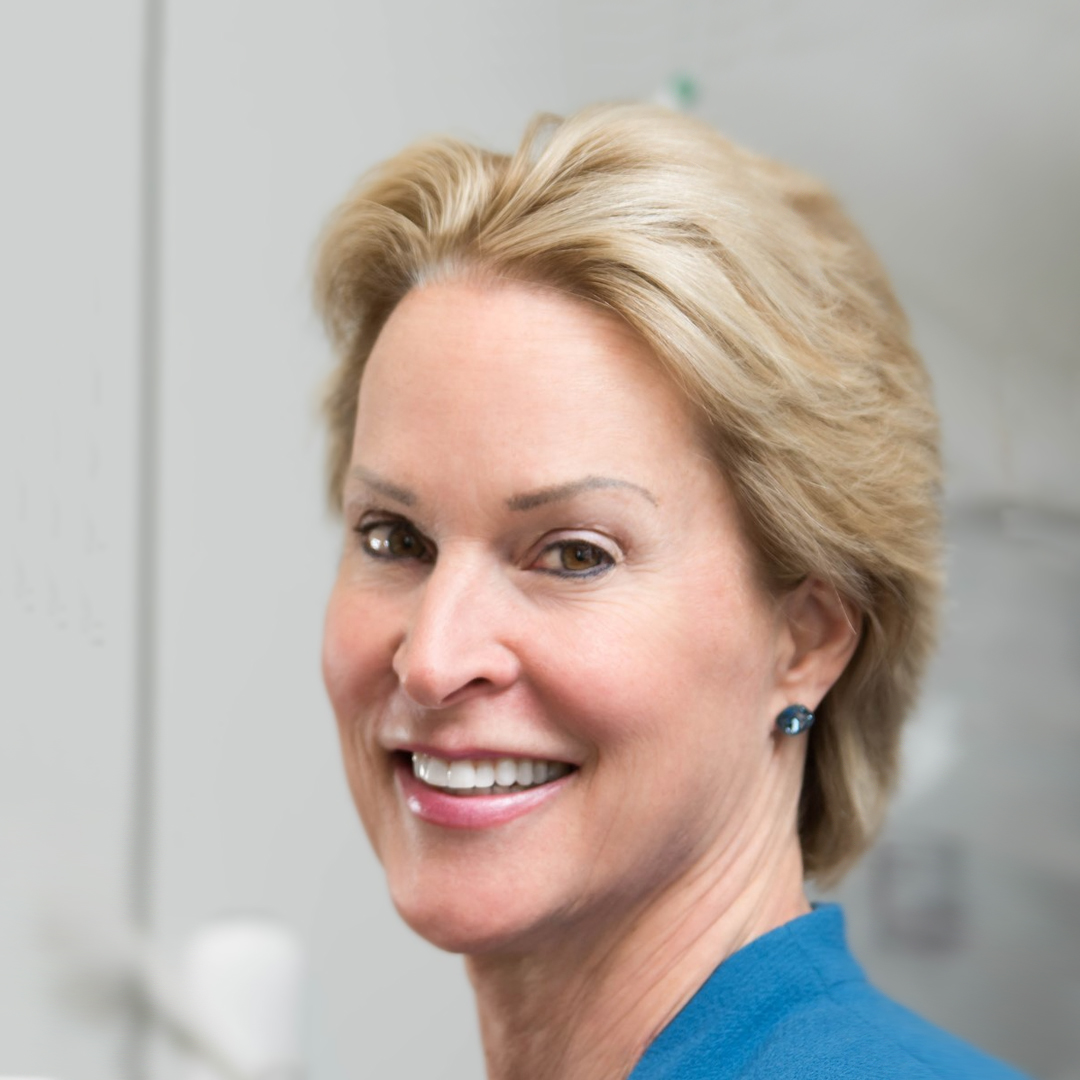
2019 Bower Science Award Theme: Green Chemistry
Biofuels, prescription drugs, medical devices—they’re all products of a global chemical manufacturing industry in which Frances Arnold is a maker of makers. To create a chemical reaction that produces, say, a lifesaving drug, chemists often rely on starter substances called enzymes. They’re complex protein molecules notoriously difficult to produce and manage in the lab. Yet nature makes enzymes all the time, easily and precisely, using DNA, optimized for just the task over millions of years of evolution. By introducing random mutations into the DNA of bacteria and mimicking evolution in commercial-like lab settings, Arnold encourages the bacteria to evolve over time into enzyme manufacturing plants, producing precision-designed enzymes resistant to conditions in commercial lab settings that typically render enzymes impractical for commercialization. Her method, called “directed evolution,” made mass production viable and opened the door to medicines, biofuels, antibodies, consumer products, gene delivery systems, vaccines, and a world of products previously difficult, if not impossible, to make. Today, the Nobel Prize-winner and others who use the techniques she pioneered are shaping new solutions to the world’s grand challenges through more sustainable, less expensive chemical manufacturing.
Frances Hamilton Arnold, Linus Pauling Professor of Chemical Engineering and Biochemistry at the California Institute of Technology, loves enzymes. These marvelously complex protein molecules convert sunlight, sugar, and carbon dioxide into life. In nature, enzymes have been optimized to work in different environments over millions of years of evolution. Appreciating their importance in daily life—enzymes are used in everything from laundry detergents and beer to pharmaceuticals and fuel—Arnold has committed herself to developing new enzymes that are specifically intended for human use. Her scientific achievements are based on a wild premise: that humans can use the same principles of biological evolution, but in a practical time frame, to design sophisticated tools for industrial chemistry.
Enzymes are notoriously difficult to produce and manage in the laboratory setting, often causing delays in the development of new products. But the idea that enzymes could be made to fit the laboratory environment rather than tailoring the lab to accommodate each enzyme was entirely new—and not readily accepted. Some argued that what does not exist in nature simply can’t exist at all. Others targeted Arnold’s credentials—she earned degrees in mechanical and aerospace engineering at Princeton before earning a Ph.D. in chemical engineering from the University of California, Berkeley—and accused her of “practicing chemistry without a license.”
But Arnold was undaunted. Overcoming odds had long been par for the course: Arnold grew up in Pittsburgh, the daughter of a nuclear physicist and a homemaker, and the only girl among four rowdy brothers. Though she was not a stand-out student in high school, while living on her own and working nights as a waitress, Arnold was admitted to Princeton University. Not many women attended Princeton at that time, and almost none enrolled to study mechanical and aerospace engineering. Going against the flow has long been part of Arnold’s process.
Over the last three decades Arnold doubled down on her hypotheses, and the resulting body of work has contributed to a major paradigm shift in biological design philosophy. She is a pioneer in directed evolution methods to engineer biocatalysts for the use of living, biological systems or their parts to speed up chemical reactions and impart the desired very high chemical specificity characteristic of natural enzymes. All of this is based on the premise that artificial selection can be used in the lab setting to optimize biological function.
Directed evolution technology demonstrated that artificial selection, as practiced by breeders of plants and animals for thousands of years, could be mimicked in the lab to make new proteins. Introducing random mutations into the DNA encoding an enzyme and forcing bacteria to make the mutated enzymes, Arnold then selected those having the traits she wanted. Repeating the process on DNA extracted from the bacteria with the best enzymes, Arnold was able to “breed” enzymes that, while perhaps irrelevant in nature, are essential to human use.
For example, naturally occurring enzymes often lack features necessary for commercial applications, such as the ability to stay active in the presence of organic solvents and in relatively high concentrations. By generating many variants and selecting the best ones, Arnold directs the evolution of viable enzymes, opening the door to a world of products that were previously difficult or impossible to produce.
Another significant example of the capabilities of Arnold’s technologies is her creation of a large set of new cytochrome P450 enzymes. The flexible structure of these enzymes gives them a wide range of functions in nature; in humans, cytochrome P450 enzymes are involved in the metabolism of hormones, vitamins, and toxins. Using directed evolution, Arnold has built on this flexible backbone to create novel catalysts that perform transformations unknown in the biological world, but important for a variety of chemical and pharmaceutical purposes. In addition to these applications, Arnold’s work gives scientists a fundamental understanding of how proteins evolve and function in nature.
Importantly, Arnold’s technologies provide a means of replacing traditional catalytic chemistries with processes that are more environmentally friendly. Though synthetic chemistry makes possible many of the products we rely on every day, these chemical processes are often derived from petrochemicals, depend on rare or toxic compounds, or generate large amounts of waste. Arnold sees her experiments with biological evolution as a source of cleaner, better chemicals. Of note, Arnold and two of her students founded the company Gevo in 2005; it is now a publicly-traded company producing isobutanol, a leading candidate biofuel that could serve as a replacement for or an additive to fossil fuels. Isobutanol will undoubtedly be of increasing interest as the search for renewable resources to replace fossil fuels becomes ever more urgent.
These days, Arnold’s critics have little to say. Her groundbreaking advancements have led to many honors, including her recognition as one of a small handful of researchers elected to all three branches of the U.S. National Academies (Science, Engineering, and Medicine). In 2018, she became only the fifth woman—and the first American woman—to receive the Nobel Prize in Chemistry. Arnold is a singular talent. Her insight, intuition, and tireless work have provided unique and powerful contributions to the chemical industry, shaping a new responsibility for preserving the sustained health of our planet.
Information as of March 2019