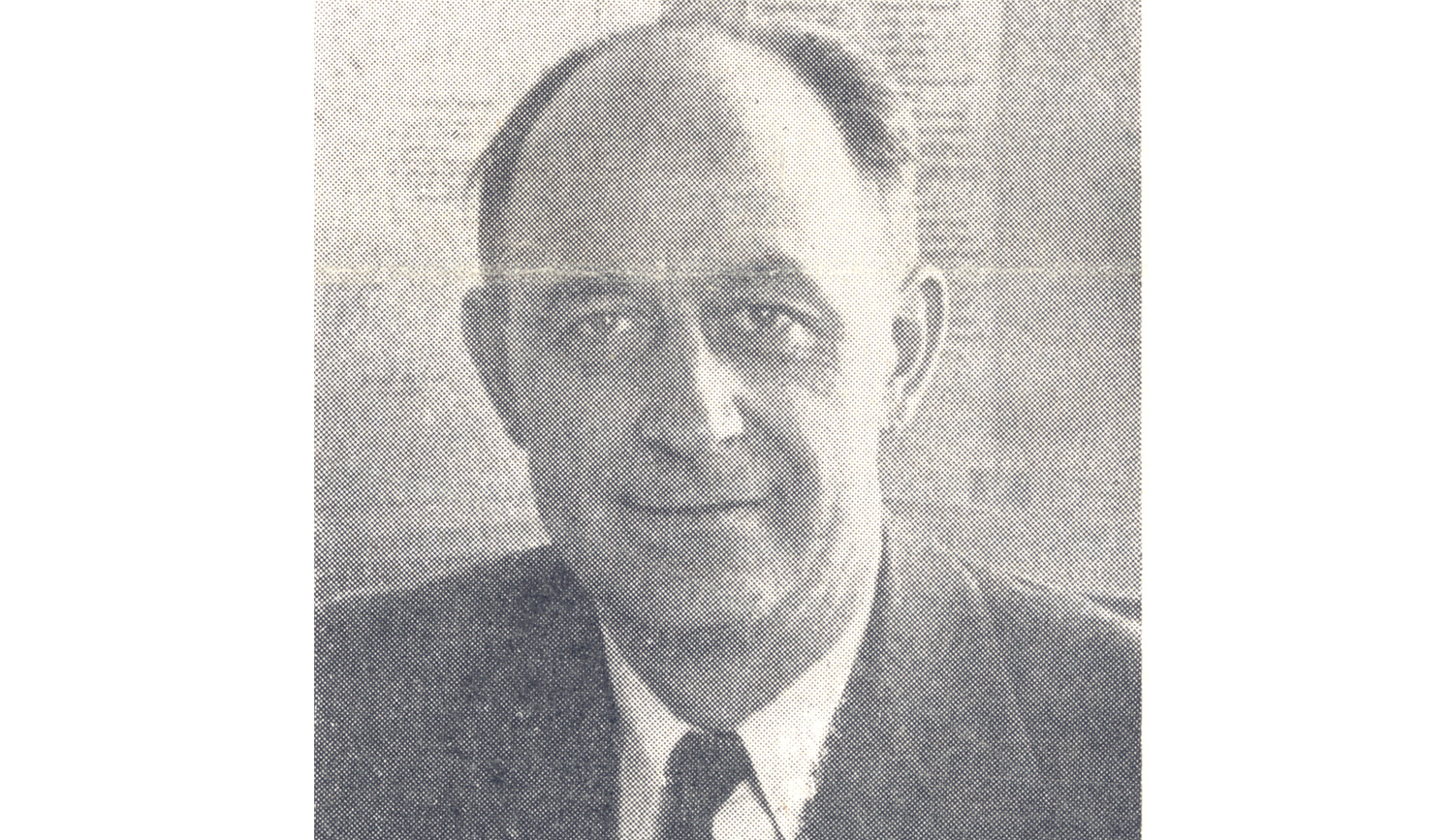
Introduction
Born in 1901, Enrico Fermi was truly a twentieth century scientist. The story of his childhood, education, and career seems familiar and "modern." Like many brilliant scientists of his time, Fermi saw events occurring in Europe as an added incentive to work in America. Europe's loss was America's gain. In New York and Chicago, Fermi found the environment and technology that he needed to advance and prove his theories. By 1947, Fermi was a celebrated scientist, known worldwide for his advances in theoretical and experimental physics.
Who was Enrico Fermi? What were his contributions to theoretical and experimental physics?
An Exceptional Student
Enrico Fermi was born September 29, 1901, in Rome, Italy; he was the youngest of three children of Alberto Fermi, a railroad official, and Ida de Gattis, an elementary teacher with firm expectations.
The death of his brother, Giulio, in 1915 during minor surgery was a crushing blow to the family. While his mother was deeply grieving, Enrico filled the emptiness he felt with study. Reading physics and mathematics texts became his hobby. His wife, Laura Fermi, once described Enrico telling her how he used to sit on his hands to keep warm at home while studying and how he would "turn the pages of his book with his tongue."
A colleague of his father, Ingegner Amidei, encouraged young Enrico in his studies and directed him to the Reale Scuolo Normale Superiore, a subsidiary of the University of Pisa, which specifically targeted promising and talented students and admitted them through competitive examinations. When Enrico Fermi submitted an essay on vibrating strings that amazed the examining professor, he was admitted to the school and was declared "exceptional." So, at 17, he moved from Rome to Pisa, the site of Galileo's famous experiments hundreds of years earlier.
While at the University of Pisa, Fermi advanced in his theoretical approach to spectroscopy. He obtained a doctorate from the university in July 1922 with his thesis on the investigation of X-rays.
Fine Young Scientist
Back in Rome, Fermi made the acquaintance of Orso Mano Corbino, the head of the University of Rome's engineering school and a senator in the nation's government. The Italian government granted Fermi scholarships and fellowships which permitted advanced studies with two specialists in quantum mechanics: Professor Max Born, the Physics Nobelist, at the University of Gottingen and Dr. Paul Ehrenfest at the University of Leiden. Werner Heisenberg was a classmate at Gottingen.
Returning to Italy in 1924 as Mussolini's Fascist government was forming, Fermi, still a protégé of Corbino, took the specially created position as Chair of Theoretical Physics at the University of Rome. Corbino's aim was the restoration of Italy's diminished scientific standing and he began recruiting the finest young scientists to build Fermi an outstanding physics department.
At first, Fermi's group worked with spectroscopic phenomena and quantum mechanics. In this time, before the discovery of the neutron and while quantum theory was still being developed, Fermi focused his attention on the properties of electrons. His theory of Fermi Statistics concerns the probability of electron distribution at given levels within the atom. He determined that his namesake fermions, the basic sub-atomic particles of matter, obey the Pauli Exclusion Principle.
Laura Capon, a chemistry student, and Enrico Fermi were married in 1928 and their children, Nella and Giulio were born in 1931 and in 1936.
Fermi first visited the United States in 1930 to address a summer symposium on quantum theory; he returned in the summers of 1933 and 1935 through 1937.
Interested in learning more about Enrico Fermi? Learn More About His Benjamin Franklin Award
From Theoretical to Experimental
Following the 1932 discovery of the neutron and then the 1934 discovery of artificial radioactivity, Fermi resolved to experiment in producing artificial radioactivity by substituting neutron bombardment for the alpha particles method the French scientists had used. This signified a change in his academic priority from theoretical to experimental science.
He devised a procedure to produce neutrons from the combination of radon and beryllium, built a Geiger counter to measure the radioactivity produced, and began bombarding elements, proceeding systematically through the Periodic Table. The first success in detecting radioactivity came with fluorine (atomic number=9) and the most noteworthy was with uranium (atomic number=92). The disintegration of uranium produced a fleeting, unstable element of atomic number 93, never before known to exist. Immediate worldwide publicity about this "new element" followed the discovery, but Fermi considered this publicity both premature and inappropriate. He considered claims that the scientific success was due to the Fascist environment equally unjustified. The full impact of the discovery of uranium disintegration would follow some years later.
Discovery
Investigations continued and in October 1934, erratic results were noticed during irradiation experiments on silver. The resulting radioactivity of the metal depended on its placement inside the protective lead container. So experiments were set up to compare the radioactivity produced when various materials were inserted between the radon source and the silver target. On October 22, as an alternative to the heavy metal lead which showed slight activity increase, Fermi suggested a light material: paraffin wax. His accidental choice worked well. The Geiger counter showed the artificial radioactivity of this silver sample to increase by up to a hundred times—the excitement grew. Fermi's explanation was that the multiple hydrogen atoms in the paraffin effectively "slowed" the neutrons to a state that permitted many more collisions with the atoms of silver. The next amazing possibility of controlled or "harnessed" radiation exposure by neutron bombardment was near.
Foreseeing the ramifications of this discovery, Corbino insisted that a patent be applied for immediately. The application was filed on October 26, 1934.
Further work followed this momentous discovery, but the group of scientists gradually dispersed as the pace of experimentation slowed; most traveled to North America. The uncertain political climate in Italy and the likelihood of war weighed on the Fermis. With the Rome-Berlin Axis in place, a crusade of anti-Semitism began in 1938. Laura Fermi was Jewish.
Tremendous Energy
On November 10, 1938, Enrico Fermi was awarded the Nobel Prize in Physics for his "identification of new radioactive elements and his discovery, made in connection with this work, of nuclear reactions effected by slow neutrons." He had previously known of this possibility and had been in contact with American universities about employment possibilities. The Fermi family was given government permission to travel to Stockholm for the Nobel Prize presentation. They did not return to Italy. Instead, after visiting Niels Bohr in Copenhagen, they sailed on December 10 for the United States and Fermi's new professorship at Columbia University.
Meanwhile in Germany, it had been discovered that the neutron bombardment of uranium results in two products of similar atomic weight. The expectation had been one product close to uranium in the Periodic Table plus small disintegration products. The reaction now occurring was more appropriately described as fission, not disintegration.
Fermi began to understand the implications of this news and went on to hypothesize that the splitting of a uranium atom with a neutron results in the release of two neutrons. Each of these neutrons would then split another atom resulting in four neutrons, and so on. This self-perpetuating chain reaction would produce tremendous energy. Its importance was not lost on the scientific community. In an atmosphere of impending war, the possibility of new weapons of unimaginable intensity appeared.
Fermi began to test his hypothesis at Columbia University using the cyclotron there as a neutron generator; within months, Fermi's hypothesis was confirmed.
War Work
Elsewhere, the danger of war was increasing, the German scientists had experience with nuclear fission. While weapon applications of this phenomenon were remote, they existed. Such considerations led the Columbia physicists to enlist the help of Albert Einstein to inform the United States government of developments. President Roosevelt reacted by establishing an Advisory Committee on Uranium (the Uranium Committee).
By then, Britain and its colonies were at war with Germany and, six months later, with Italy, too. Fermi's research became "war work" upon the United States entering the war on December 8, 1941. He also automatically became an "enemy alien" with the complications that entailed.
On October 12, 1942 the "enemy" status was lifted for Italians; Fermi became a citizen of the United States on July 11, 1944.
Atomic Pile
At Columbia, Fermi and his team continued investigations into the feasibility of controlled chain reactions from nuclear fission. Experimentation led them to build an "atomic pile," beginning as a stack of pure graphite brick surrounding a neutron source. This first step enabled the examination of graphite's effect on neutron activity: absorption and re-emission, quantities, fissions. Step two was the addition of uranium to the experiment. The original stack was rebuilt with some of the graphite bricks being seeded with pieces of uranium. Observations on the effect of graphite resumed. Results showed Fermi that a stack larger than the current "pilot" version was needed to produce a measurable nuclear chain reaction, and a search for larger facilities began.
The expansion at Columbia was slowed by the U.S. government's decision to accelerate and centralize atomic research. Fermi's work eventually relocated to the University of Chicago in 1942. Secrecy covered all endeavors at this location, divertingly labeled the Metallurgical Laboratory. The physicists who gathered at the new facility concentrated on fundamental atomic research as an arm of the newly-named Manhattan Project, the first instance of "big science" with the research, materials production, and support personnel consolidated and directed to a single goal.
Now, Fermi had the space needed for his enlarged atomic pile. That space—about 200 sq. ft. in area and more than 26 ft. high in the unused squash court under the West Stands of Stagg Field Stadium in the middle of a city of over 3 million people—was destined for lasting fame.
The Fermi group in Chicago built and examined small piles, becoming confident that all parameters to create a pile of the critical size and composition for sustained chain reaction were known. In a period of just six weeks, the final pile, standing just less than 26 feet high and completely encased in an enormous square balloon of rubberized cloth, was built. On December 2, 1942, Fermi managed the historic operation, directing the gradual removal of the control rods and monitoring the consequent increases in radioactivity. As everything went according to plan, Fermi, a creature of habit, declared a break for lunch. Work resumed after lunch and at 3:20 in the afternoon the last control rod had been carefully withdrawn in one-foot increments when Fermi gave the final instruction to remove it completely. All monitoring instruments showed rising radioactivity—the controlled nuclear fission chain reaction had been achieved!
The message reporting success sent by the director, Arthur Compton, to the Office of Scientific research and Development said, "The Italian Navigator has reached the New World." A toast of Chianti was raised in celebration.
Meanwhile, the crash program to develop weapons incorporating this achievement had been proceeding. An atomic bomb, with an uncontrolled nuclear explosion, was envisioned.
Mr. Farmer
Fermi now traveled the country visiting sites crucial to the war effort—in Hanford, Oak Ridge, Argonne, and Los Alamos. He also merited a constant bodyguard, John Baudino, and the codename "Mr. Farmer." In the summer of 1944, the Fermi family moved from Chicago to Los Alamos. They remained there until December 31, 1945.
July 16, 1945, was the date of the first atomic bomb test at Trinity in the New Mexico desert. Fermi observed it from the base camp some 10 miles from the blast site. He described having a wide board with a dark welding glass insert to protect his face and feeling the heat sensation on exposed parts of his body.
Ever the scientist, Fermi wondered about the strength of the blast. He described the simple test he made:
"About 40 seconds after the explosion the air blast reached me. I tried to estimate its strength by dropping from about six feet small pieces of paper before, during, and after the passage of the blast wave. Since, at the time, there was no wind I could observe very distinctly and actually measure the displacement of the pieces of paper that were in the process of falling while the blast was passing. The shift was about 2.5 meters, which, at the time, I estimated to correspond to the blast that would be produced by ten thousand tons of T.N.T."1
Following the blast, Fermi examined the 800 ft. diameter crater from the safety of a lead-lined Sherman tank, noting the glazed desert surface—the sand had melted and re-solidified.
An Alternative Use
On August 6, 1945, the atomic bomb was dropped on Hiroshima and the next on Nagasaki three days later. Japan surrendered on August 14, ending the six years of World War II. Germany had earlier surrendered on May 7, 1945. While German scientists had been prominent in the discovery and identification of nuclear fission, their wartime application efforts had not succeeded.
Atomic scientists had also pointed out the peacetime use of nuclear fission as an alternative to oil and coal in providing the heat transfer energy to create steam for turbines in electricity generation. A program of nuclear power plant construction began.
Back in post-war Chicago, Fermi became a professor at the Institute for Nuclear Studies at the university. His interest now lay in the nature and origin of cosmic rays: the high-energy, high-speed particles that bombard the earth from outer space. Construction of the giant cyclotron in Chicago in 1947 gave a convenient source of sub-atomic particles for study. Fermi theorized that cosmic ray particles begin and gain their speed from collisions with clouds of magnetism in outer space. The Fermi-Walker transport process describes this condition in terms of general relativity.
Enrico Fermi died in Chicago from stomach cancer on November 28, 1954. Since then, space exploration has grown and increased, and now, cosmic rays in space are being examined directly. Hardware to artificially create cosmic rays is no longer essential, but those developing new theories stand on the shoulders of Fermi in extending knowledge of the sub-atomic particle universe.
Background
Fermi grew up during times burgeoning with new theories and ideas in atomic physics. Thomson's discovery of the electron was made in 1897; in 1910 came Rutherford's perception of the central, minute nucleus containing all the mass and positive charge of the atom; Chadwick's discovery of the neutron; then Pauli describing the behavior of the three particle types which comprise the atom: electron, proton and neutron.
Enrico Fermi, the complete scientist, combined his aptitudes for both theoretical deduction and experimentation throughout his career, beginning with the pure theory of Fermi-Dirac statistics through deductions of experimental results in neutron bombardment, successful designs of atomic piles, explanation of the final results of nuclear fission, and ending with reasons for the acceleration of cosmic rays.
Fermi-Dirac Statistics
During his postgraduate study at the University of Gottingen, Fermi developed the distinct statistical model based on the behavior of a perfect gas, predicting the actions of large clouds of electrons and showing that they have different activity from other sub-atomic particles—a fundamental characteristic of matter. Such elementary particles, the basic elements of matter, are named fermions after Fermi.
Beta Decay
"Alpha" and "beta" radiation was the description given to the newly-discovered phenomena at the beginning of research into radioactivity. More accurately, the alpha particle is the nucleus of the helium atoms having two protons and two neutrons, and the much higher energy beta particle is an electron released from the atom.
The Fermi theory of beta decay describes the probability of decay, or transition, in terms of the statistics of nuclear forces at the moment of decay. In his calculation, Fermi proposed a new particle, the neutrino, to account for the slight loss of electron energy observed during the process and preserve the principle of conservation of energy.
Twenty-five years after Fermi's theory, detailed understanding of the beta decay mechanism was reached and the theory was confirmed. This theory is also called Fermi's Golden Rule.
Slow Neutrons
Natural radioactivity was discovered by Becquerel in 1896 and characterized by Pierre and Marie Curie two years later in their isolation of polonium and radium. Artificial radioactivity was discovered some thirty years later by their daughter, Irene, and her husband, Frederic Joliot. In one experiment, they bombarded boron with alpha particles, converting some of it to nitrogen then confirming that the radioactivity from the boron transferred to the nitrogen. This method was also effective on aluminum but not on heavier elements due to the low energy of alpha particles. Alpha particles have drawbacks as "bullets." Being positively charged helium nuclei, they are slowed by electrical interference from surrounding electrons and meet resistance from the positively charged target nuclei. Their speed, power, and effective distance are diminished.
From this information, Enrico Fermi speculated that neutrons, with neutral polarity and higher speed, would make more effective "bullets" than alpha particles. His experimental approach was to move methodically through the periodic table, subjecting each element to neutron bombardment.
The first hurdle was to devise a reliable source of neutrons which are obtained from the collisions of alpha particles with certain elements. Fermi took radon from the disintegration of a radium source and mixed it with beryllium powder and sealed it in a glass tube. The tube was his neutron source. He built the Geiger counter used to measure the radioactivity results and gathered chemical procedures to separate and identify the elements created by disintegrations.
Fluorine, the ninth, was the first element to show radioactivity from neutron bombardment; the aim was to examine as many as possible of all 92 naturally occurring elements in the periodic table.
The method was to bombard a sample, measure the resulting radioactivity, chemically separate the irradiated sample, and measure the radioactivity of each separated element. It was shown that the element present after disintegration was close in atomic number to the original target sample. This outcome held true until the target sample was uranium; the post-bombardment mixture contained a number of elements, including one of atomic weight 93. A new element—even an unstable one—had apparently been created.
The team's next breakthrough came when a scientist noticed that placement of the sample and objects around it influenced the radiation outcome. Intrigued by this, the team began a new path of investigation, varying the materials between the neutron source and a silver target and measuring the resulting radioactivity.
During the experiments Fermi suggested trying a light material, such as paraffin wax, be used as the intermediate material around the neutron source in place of the heavy metal, lead. The results were astonishing—the silver's radioactivity increased a hundredfold. Fermi's theory from these results introduced the slow neutron concept.
Paraffin wax, a solid mixture of hydrocarbons, contains a high percentage of hydrogen atoms. The nuclei of these atoms, single protons, are equal to neutrons in mass. When neutrons enter the wax, the high hydrogen content assures a large number of collisions and the similarity in particle size slows the neutrons' speed when collisions occur. The "slow" neutrons striking the target will be more likely to collide with silver atoms; the increased collisions result in higher radioactivity.
In these experiments, the attention of Fermi's team was on testing for periodically adjacent elements from decomposition rather than more distant elements in the periodic table. The possibility of other decomposition products went unnoticed. It was left to Hahn, Strassman, and Meitner, two years later, to discover that the irradiation of uranium causes nuclear fission. The inference was that the new element thought to have been discovered was in fact a mixture of uranium's decomposition products.
Chain Reaction
On learning of the nuclear fission discovery, Fermi, working with Szilard, immediately added a new hypothesis to his list. His tentative explanation for the high energy generated by the uranium atom on splitting assumed that the original single atom causing the split produced two neutrons. It would be possible that these two would then collide with other uranium atoms and produce four neutrons, and so the chain of reactions would grow as would the number of neutrons. The process would continue until all uranium atoms were used up. Each nuclear collision releases huge amounts of energy.
Fermi's hypothesis described an ideal condition. It had to be acknowledged that in real situations, energy production and reaction rates would be reduced by missed collisions due to high nuclear speed and slowed interaction from intra-nuclear absorption, as Fermi had already discovered in his paraffin wax experiments.
Experimental confirmation required a reliable neutron source, a suitable vessel to house the chain reaction, and a method of controlling, or moderating, the reaction rate. The neutron source was created by bombarding beryllium with highly accelerated deuterons (nuclei of deuterium atoms) created in a cyclotron. The vessel was a room-size pile of ultra-pure graphite seeded with a lattice of uranium and the moderators were removable graphite rods inserted at intervals in the pile.
The chain reaction occurs at critical mass, the point at which there are just sufficient neutrons to sustain the reaction after accounting for neutron loss from the pile due to escape and absorption. The pile that provided the first chain reaction was a spherical pile almost 26 ft. in diameter.
Credits
The Enrico Fermi presentation is made possible by support from The Barra Foundation and Unisys.
This website is the effort of an in-house special project team at The Franklin Institute, working under the direction of Carol Parssinen, Senior Vice-President for the Center for Innovation in Science Learning, and Bo Hammer, Vice-President for The Franklin Center.
Special project team members from the Educational Technology department are:
Karen Elinich, Barbara Holberg, and Margaret Ennis.
Special project team members from the Curatorial department are:
John Alviti and Andre Pollack.
The project's Advisory Board Members are:
Ruth Schwartz-Cowan, Leonard Rosenfeld, Nathan Ensmenger, and Susan Yoon.
Read the Committee on Science and the Arts Report on Enrico Fermi’s early life and his work in the fields of theoretical and experimental physics.