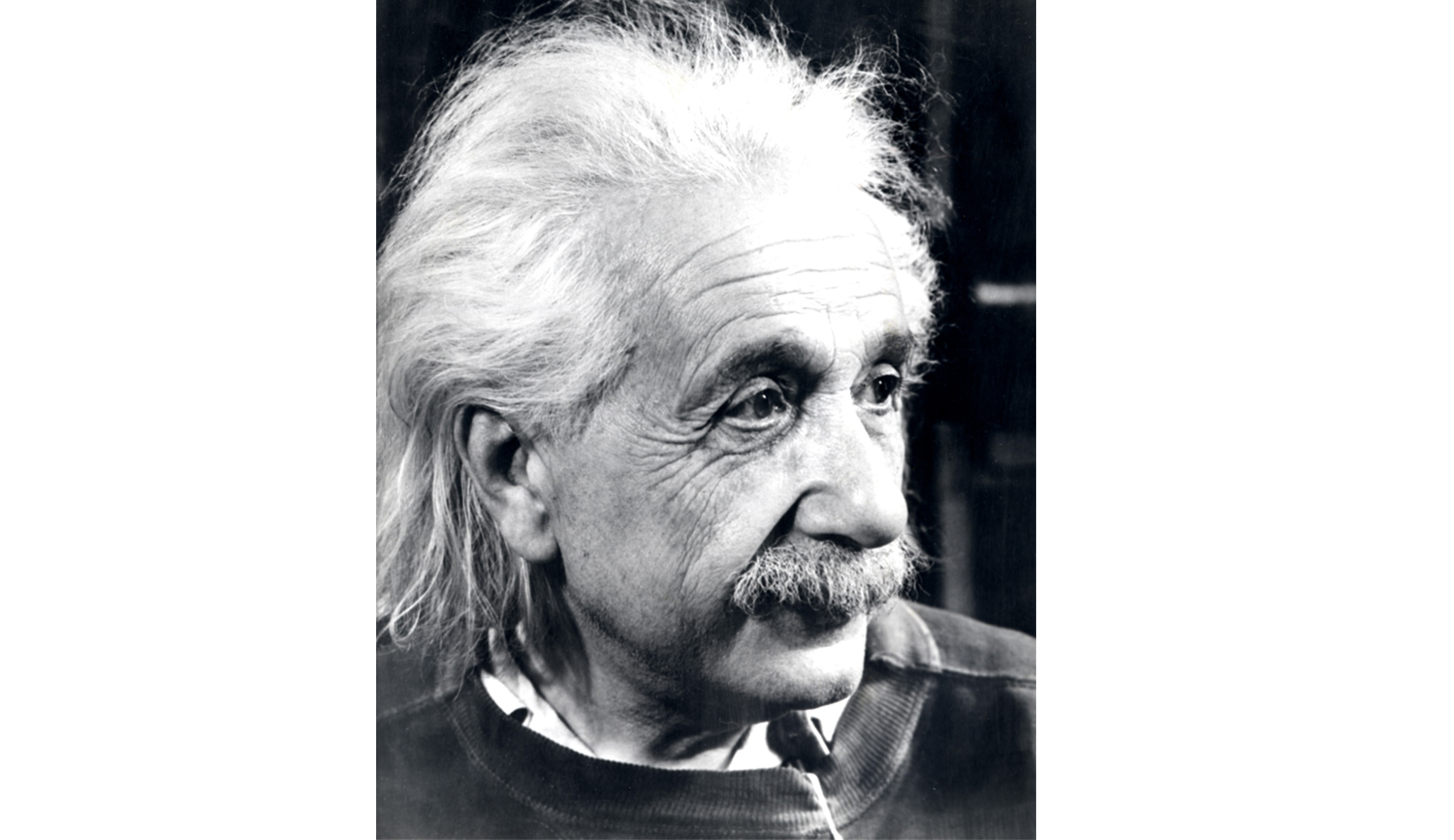
Introduction
Though he described himself as a "mathematical ignoramus," Albert Einstein's thinking was so complex that accomplished members of the scientific community still struggle to wrap their minds around the meaning and implications of his theories. Born in Germany in 1879, the frizzy-haired physicist conducted some of his most important research in Princeton, New Jersey, where he spent the later years of his life. Perhaps best known for his Theory of Relativity and his equation E=mc2, Einstein's work revolutionized the field of theoretical physics and made him a celebrity throughout the globe.
As he presented Einstein at Medal Day exercises, Dr. Frederick Palmer, Jr. of The Franklin Institute's Committee on Science and the Arts said:"The romance of his achievement has been such that mathematical physics has become popular with the public."
Who was Albert Einstein? What were his achievements in the field of physics?
The Nature of a Genius
Before he was known as a genius whose work profoundly changed the way the world thinks about physics, Albert Einstein thought of himself as "merely curious." In his youth, his curiosity lead him to explore the field of natural science through private reading outside of his high school classes, and to apply his knowledge to his own thoughts and questions about the nature of the cosmos.
Einstein was a philosopher and a human rights activist as well as a scientist. During his lifetime he witnessed two world wars and predicted the invention of the atomic bomb in a now-famous letter to President Franklin Delano Roosevelt. Einstein eloquently recorded his thoughts on religion, science and human rights, and the pages of his writings are imbued with the complex emotions and musings of a man who witnessed profound changes in the world around him, and whose direct involvement in major scientific breakthroughs inspired him to think about the extent to which developments in science effect society at large.
Despite the fame brought to him by his theories and research, Einstein's sense of humility remained intact. Though anecdotal episodes from his youth show some signs of arrogance and frustration with his fame, his adulthood is marked by a mature gratitude for his abilities and a resigned acceptance of his celebrity status. Reflecting on his success in his later years Einstein wrote, "For the most part I do the thing which my own nature drives me to do. It is embarrassing to earn so much respect and love for it."
The "Lone Traveler" Sets Out
Albert began his schooling in Germany, where his teachers disciplined him and his classmates were disrespectful to the young Einstein. His primary school classes emphasized memorization and learning by rote. Albert was reprimanded by his German elementary school teachers for thinking too much about the meaning of their questions and failing to produce responses as quickly as his peers. At home, Albert obediently completed his homework before engaging in solitary games. One of his favorite pastimes as a child was constructing houses of cards, which sometimes was able to reach four stories. Even as a young child Einstein valued solitude, and in 1930 he would reflect: "I am truly a 'lone traveler' and have never belonged to my country, my home, my friends, and even my immediate family with my whole heart; in the face of all these ties, I have never lost a sense of distance and a need for solitude—feelings which increase with the years" (qtd in Cassidy 64).
Working Ahead
In the fall of 1888, when Einstein was nine years old, he entered a secondary school in Munich, Germany called the Luitpold-Gymnasium. This school emphasized non-scientific subjects like Latin and ancient Greek. While he did earn good grades in his classes, they did not spark his interest. It was during these secondary school days that Albert began to diverge from the curriculum prescribed for him, engaging in his own private reading. At age thirteen he asked his parents to purchase the mathematics textbook that he would be using the following year, and proceeded to work his way through the entire mathematics program at the Lutipold-Gymnasium in a matter of months. He indulged his passion for physics and physical phenomena by reading textbooks that were, at the time, key writings on the natural sciences.
In Need of a Liberal Arts Education
As his thoughts shifted towards college and more advanced studies, Albert was determined to apply to the Federal Institute of Technology (FIT) in Zurich, Switzerland. He disliked the Lutipold-Gymnasium and did not complete his studies there. He instead committed himself to a period of self-study, during which he acquired knowledge of theoretical physics. He took the FIT's competitive entrance exam at age sixteen, more than a year younger than the other students who sat for the exam at the same time. The results of his exam revealed that he had done well on the mathematical-physical section of the test, while he had failed the general portion of the exam which tested his knowledge of literary and political history and of foreign language. Albert was thus required to attend a secondary school in the nearby Swiss town of Aarau before he was admitted to the FIT.
Einstein began his studies at the Federal Institute of Technology (FIT) in October of 1896. As a college student he often skipped lectures and studied for tests by borrowing notes from his classmates, and would later describe himself as a mediocre university student. While not an avid participant in his classes, Albert's genuine interest in theoretical physics inspired him to devote large periods of time to its study. He participated in a number of physics experiments while a student, and consistently strove to unite the abstract concepts of theoretical physics with practical matters. His doctoral thesis made strides towards such unification, combining the theoretical claim for the existence of molecules with a description of the physical law governing the behavior of molecules. Einstein used experimental data to further describe this law and to further develop the relationship between the theoretical and the practical.
Princeton Days
After he completed his degree at the FIT, Einstein found work as an assistant professor and eventually as a full professor of theoretical physics. He preferred researching to teaching, and in 1914 he accepted a paid research position in Berlin, Germany, which was considered the "capital city" of physics at that point in time. In 1933 the rise of Nazi power in Germany prompted Einstein to resign from his position in Berlin and flee to the United States, where he took up residence at 112 Mercer Street in Princeton, New Jersey and assumed a position on the faculty of Princeton's Institute for Advanced Study.
Oswald Veblen, the first professor in the Institute for Advanced Study, helped select and relocate Einstein and other foreign mathematicians after Hitler's rise to power in Europe. Veblen was a leading geometer and served a term as president of the American Mathematical Society and of the International Congress of Mathematicians, held at Harvard. Though highly respected as a scholar, Veblen valued his relationships with his students and helped design common spaces in Princeton buildings in order to help encourage the formation of student-faculty relationships.
The verification and publication of Einstein's Theory of Relativity in 1919 brought him instant celebrity status.
Under Investigation
In August of 1939 Einstein mailed a letter to the White House, informing President Franklin Delano Roosevelt of the potential threat posed by the discovery of and subsequent experimentation with nuclear fission in Berlin, Germany. His ominous prediction read:
"This new phenomenon would also lead to the construction of bombs, and it is conceivable—though much less certain—that extremely powerful bombs of a new type may thus be constructed. A single bomb of this type, carried by boat and exploded in a port, might very well destroy the whole port together with some of the surrounding territory."
History indicates that Einstein sent four letters to President Roosevelt, each expressing an increased urgency for action. In December of 1941, Roosevelt heeded Einstein's warning and convened the American investigation into nuclear fission and the development of such a bomb known as the Manhattan Project. This top secret project went underway in a laboratory in Los Alamos, New Mexico. Four years later, in 1945, the United States dropped the newly-developed atomic bomb, devastating the Japanese cities of Hiroshima and Nagasaki.
Despite his role in alerting the President to the possibility of nuclear weapons, Einstein did not participate in the Manhattan Project. Though he was granted American citizenship in 1940, his involvement with liberal organizations whose missions called for world peace made Einstein a "radical" in the eyes of the Federal Bureau of Investigation. In response to the perceived threat posed by Einstein, the FBI compiled an extensive secret file on the scientist, monitoring and recording his movements. His status as a security threat prevented Einstein from gaining the security clearance necessary to enter the secret laboratory in New Mexico. It is very likely that this was not a source of disappointment for Einstein, who publicly declared his dedication to pacifism. He was quite distressed when the public mind associated him with the dropping of the atomic bombs in 1945 and the subsequent civilian casualties.
Interested in learning more about Albert Einstein? Learn More About His Benjamin Franklin Award
Personal Commitments
Einstein committed to his family, and throughout the course of his life he married twice and had three children. All three children were a product of his relationship with Mileva Maric, whom he encountered while he was a university student. Mileva was a classmate and a fellow scientist, and evidence suggests that she was instrumental in the development of some of her husband's theories. Einstein's children were named Lieserl, Hans Albert and Eduard, who was known as "Tete." Einstein eventually divorced Mileva, marrying his cousin Else Löwenthal four months later.
Einstein was also deeply committed to his Jewish faith. His religious beliefs inspired him to grapple with philosophical thoughts and to champion the cause of Zionists and their quest for a Jewish home state in Palestine. He was offered the presidency of Israel in 1952, though he declined this honor. He died three years later of an aneurysm of the abdominal aorta, bequeathing much of his writings and photographs to the Hebrew University of Jerusalem.
An Eternal Riddle
Though he is conceived of as a genius in modern society, Einstein's ways of thinking diverged sharply from those of a majority of other scientists when he initially penned some of his most famous theories. In the early years of the 20th Century, theorists were not regarded with great respect, but Einstein viewed theoretical work as a high calling. Contemplating theoretical physics, Einstein wrote, "I soon learned to scent out that which was able to lead to fundamentals and to turn aside from everything else, from the multitude of things which clutter up the mind and divert it from the essential...Out yonder there is this huge world, which exists independently of us human beings and which stands before us like a great, eternal riddle."
Electromagnetic Waves
Some scientists in the late 1800s and early 1900s believed in and described an entity known as "the ether." The ether was thought to be a backdrop at a state of absolute rest against which the movement of elements of the cosmos occurred. Einstein disagreed with the existence of the ether, which will be seen during the discussion of his theory of special relativity. However, an understanding of the ether is important for understanding the theory of electromagnetic phenomena which preceded Einstein's theory of relativity.
During the 19th Century, scientists Michael Farady, James Clerk Maxwell and Heinrich Hertz formulated a theory that described electromagnetic phenomena. This theory indicated that electric and magnetic forces resulted from the effect of electric and magnetic fields existing in space between electric charges. These electric charges were produced by the ether, which was thought to be able to exert electric forces on ordinary matter. Hertz showed that moving electromagnetic fields could break away from ordinary matter and propagate through the ether as independent electromagnetic waves carrying energy. These electromagnetic waves come in both visible and invisible forms. Hertz showed that visible light is one visible form of the electromagnetic wave. Invisible electromagnetic waves include radio waves, x-rays and microwaves. The concept of such waves moving through the ether can be likened to the waves that spread over a pond after a stone is thrown into the water. The ripples in the pond can be thought of as the equivalent of electromagnetic waves, and the still water as the equivalent of the ether. In a pond, the force of the stone hitting the water results in the ripples. One of the things puzzling the scientists of Einstein's time was what exactly caused the formation of electromagnetic fields whose independent movement resulted in the electromagnetic waves which they conceived of as moving through space.
The Electron
In 1897, the source of electromagnetic fields was discovered: the electron. At the time of its discovery, the electron possessed the smallest mass known. It also carried the smallest electric charge known. Because of its charge, it was found to be the source of electromagnetic fields. However, the electron posed a problem for scientists grappling with electromagnetic theory. As is discussed above, electromagnetic theory dealt with fields and waves, entities that were thought to be continuous and without mass. Electrons are neither continuous nor without mass: they are individual, charged particles that have mass. Electrons thus did not "fit into" electromagnetic theory as it was understood in the late 19th Century. They posed yet another riddle for Einstein and his contemporaries.
A Quantum Leap
In 1905, Einstein challenged the concept that visible light, one form of the electromagnetic wave, always behaved as a continuous wave. Einstein argued that in certain cases light behaves as individual particles. He called these particles "light quanta," and said that each "light quanta" carries a "quantum," meaning a fixed quantity of energy. A light beam is thus composed of many "light quanta" which are observed as one continuous wave. The total energy of a light beam, Einstein said, is the sum total of the individual energies of the distinct "light quanta." Today, these "light quanta" are called "photons." Theories that treat total energy as "quantized" (meaning that total energy is calculated by adding together the fixed energies of the individual "quanta" of which the overall energy is composed) are known as quantum theories.
It's (Photo) Electric!
Einstein's light quantum hypothesis helped to explain certain visible light behavior which could not be explained if visible light were understood to exist in the form of a wave, rather than in the form of tiny individual particles. One of these phenomena was known as the photoelectric effect. Scientists had observed that, when light hit metal, electrons were ejected from the surface of the metal. Einstein's light quanta could eject electrons from the surface of the metal by changing the energy states of the electrons they hit. Light quanta are little bundles of energy, and according to electron theory, electrons absorb energy. The act of absorbing energy takes an electron to a higher energy state, causing it to jump. When it returns to its state of rest, it emits the energy it has absorbed in the form of light. This results in the observable ejection of electrons from the metal's surface known as the photoelectric effect.
Galileo and Relativity
Though Einstein is the scientist most frequently associated with the theory of relativity, there are several thinkers who are responsible for its formulation. The first known person to theorize about relativity was Galileo, who articulated the first "relativity principle" in the seventeenth century. In generating his relativity principle, Galileo removed the distinction between stationary and moving observers, arguing that people on earth cannot tell if they are really at rest or if they are moving with the rotation of the earth each day. To demonstrate this, Galileo used the example of a cannonball falling from the top of a ship's mast. He noted that the cannonball will land at the base of the mast whether the ship is moving steadily through the ocean, or whether it is at rest in a dock. Even if they observe the falling ball, people on the ship cannot tell if they are really at rest or if they are moving with the ship. They cannot distinguish their state of rest from the ship's state by observing motion that takes place within the "reference frame" of the ship. In other words, a person at rest on the deck of a ship cannot determine whether the ship is at rest or moving at a steady speed through the ocean by observing actions that happen on the ship itself. That person must observe the ship relative to its surrounding environment in order to make such a determination.
A Matter of Principle
In 1905, Einstein wrote a paper entitled, "On the Electrodynamics of Moving Bodies." This paper served as the foundation for his theory of relativity. It also included many of the theories and results of scientists whose work had preceded Einstein, so much so that many of his contemporaries had a difficult time distinguishing Einstein's "theory of special relativity" from other accepted theories of the time. The main difference between Einstein's theories and other prevalent scientific theories of the 1900s lies in how Einstein went about deriving his theories. While many of his contemporaries drew "constructive theories," Einstein drew "principle theories."
Einstein's theories were not hypotheses built on data reached through experimentation. Rather, they were universal principles intended to impact all of physics. Throughout his life, Einstein was driven by a desire to isolate a single theory that would unify gravitation and electromagnetic fields. Though this single theory has not yet been found, Einstein's work has inspired physicists of today to continue the search for a unified theory.
Special Relativity
Einstein's theory of special relativity is fundamentally a theory of measurement. He qualified the theory as "special" because it refers only to uniform velocities (meaning to objects either at rest or moving at a constant speed). In formulating his theory, Einstein dismissed the concept of the "ether," and with it the "idea of absolute rest." Prior to the generation of Einstein's theory of special relativity, physicists had understood motion to occur against a backdrop of absolute rest (the "ether"), with this backdrop acting as a reference point for all motion. In dismissing the concept of this backdrop, Einstein called for a reconsideration of all motion. According to his theory, all motion is relative and every concept that incorporates space and time must be considered in relative terms. This means that there is no constant point of reference against which to measure motion. Measurement of motion is never absolute, but relative to a given position in space and time. Returning to Galileo's cannonball, Einstein considered this: the cannonball falling from the mast of the ship would appear to an observer standing on the deck of that ship as though it dropped straight down; however, to an observer standing on the shore, the cannonball would appear to follow a curved trajectory on its way to the base of the mast. Which trajectory did the ball actually follow? According to Einstein's theory of special relativity, the answer is, both—and neither. Each observer's observation is valid in its own reference frame, yet each is no more than an artifact of the measurement, or observation, undertaken by the observer.
Implications of Relativity
Einstein's theory of special relativity has many complex consequences, which confuse even scientists of the present. One of the most famous consequences of this theory is the formula E=mc2. This theory relates energy to mass times the square of the speed of light. Often considered the "speed limit" of the universe, the speed of light is equivalent to about 186,000 miles per second.
Four-Dimensional Space
In 1904, mathematician Hermann Minowski succeeded in representing Einstein's theory of special relativity mathematically. He did so by introducing the concept of four dimensions: three of space and one of time. Using his mathematical representation, he was able to describe the positions and motions of objects such as speeding electrons as they moved through space. Minowski's four-dimensional space-time helped Einstein to develop his theory of general relativity, which he would come to regard as his greatest achievement.
Principle of Equivalence
Special relativity applies only to cases in which objects are moving at a uniform velocity. General relativity, however, is applicable to all forms of accelerated motion. This theory of general relativity arose from Einstein's principle of equivalence. Einstein formulated this principle by examining a given mass in two different states. The first state occurs when the mass in question is acted on by gravity, and the second when the mass is in a state of inertia (when it resists forces and accelerations). According to Einstein's principle of equivalence, the given mass is equivalent in both states. Take, for example, a spinning top. According to the principle of equivalence, the top has the same mass whether it is falling off a desk (being acted on by gravity) or whether it is spinning atop a desk (in a state of inertia). This principle may seem obvious, and in fact people since Newton's time had simply assumed it to be true. However, the implications of the principle of equivalence are far from obvious, and Einstein was the first to realize those implications.
General Relativity
Einstein's theory of general relativity unites his theory of special relativity with the concept of gravity conceived of by Sir Isaac Newton. Einstein's key insight was that gravitation is not the result of a force. It is rather a manifestation of curved space and time. Einstein's theory of general relativity can be understood by considering the following scenario. An astronaut sitting in a space capsule waiting to launch at Cape Canaveral feels his normal weight. While in space, free from gravitational pull, the astronaut feels weightless. However, if the space capsule were to accelerate upwards in space at exactly the acceleration of gravity back on earth, the astronaut would be pushed into his seat with a force exactly equivalent to his own weight. The astronaut would be unable to distinguish between the sensation of sitting in the space capsule prior to launch in Cape Canaveral, and the sensation of sitting in the space capsule as it accelerates upwards in space at exactly the acceleration of gravity. He could only distinguish between the two by looking out the window.
Curved Space
Einstein's theory of general relativity describes space as curved, with the "curved space" being the four-dimensional space-time conceived of by Minowski. The curvature of space results in the effects of gravity. This notion of curved space becomes more tangible by thinking again about the astronaut and the space capsule, but this time introducing a beam of light into the capsule. If a beam of light is shone from the top of one capsule wall to the opposite wall while the capsule is accelerating upwards in space, the light will appear curved. This is because, in the time it takes for the light beam to move across the cabin to the opposite wall, the cabin will have accelerated upwards and the beam will appear to curve across the cabin and hit below the spot directly across from where it started. The light will also appear to curve across the top of the space capsule if the capsule is at rest in Cape Canaveral. In other words, the light beam acts as if it is being pulled down by gravity. The space-time through which it moves can be understood to be curved by the presence of a massive body: in this case, the earth. In space, the curvature of space itself causes all objects, such as light or planets or spaceships, to follow the curvature. In both cases, the gravitational effect occurs because of the curvature of space.
Acknowledgement
Albert Einstein was awarded a 1935 Franklin Medal by The Franklin Institute "In recognition of his contributions to theoretical physics, especially his work on Relativity and the Photo-Electric effect."
Additionally, Einstein received honorary doctorate degrees in science, medicine, and philosophy from many European and American universities. He was awarded Fellowships or Memberships of all the leading scientific academies throughout the world.
A few of Albert Einstein's notable prizes include the 1921 Nobel Prize in Physics, the 1925 Royal Society Copley Medal, and the 1929 Max Planck Medal.
Fan Mail
Many inquisitive schoolchildren mailed letters to Albert Einstein, asking the famous physicist questions about science and about his personal life. Einstein's letters to and from children are bound together in a book entitled Dear Professor Einstein, and the abbreviated text of one of these exchanges is reproduced below. You can follow the example of the children who sent their questions to Dr. Einstein by writing to your own favorite scientists. Talk to your teacher about the kinds of questions you could ask, and how you should go about contacting the scientists whose work is of interest to you.
Cape Town, South Africa
10th July, 1946
Dear Sir,
I am awfully interested in Science, so are quite a lot of people in my form at school. My best friends are the Wilson twins. Every night after Lights Out at school, Pat Wilson and I lean out of our cubicle windows, which are next to each other, and discuss Astronomy, which we both prefer to anything as far as work goes. Pat has a telescope and we study those stars that we can see. We usually have to creep past the prefect's room to other parts of the building to carry on our observations. We have been caught a few times now, though, so it is rather difficult.
What worries me most is How can Space go on forever? I have read many books on the subject, but they all say they could not possibly explain, as no ordinary reader would understand. If you do not mind me saying so, I do not really see how it could be spiral. But then, of course you obviously know what you are saying, and I could not contradict!
I trust you are well, and will continue to make many more great Scientific discoveries.
I remain,
Yours obediently,
Tyfanny
----------
August 25, 1946
Dear Tyfanny,
Thank you for your letter of July 10th.
Be not worried about "curved space." You will understand at a later time that for it this status is the easiest it could possibly have. Used in the right sense the word "curved" has not exactly the same meaning as in everyday language.
I hope that yours and your friend's future astronomical investigations will not be discovered anymore by the eyes and ears of your school-government. This is the attitude taken by most good citizens toward their government and I think rightly so.
Yours sincerely,
Albert Einstein
View Einstein's Case File Bibliography
The Albert Einstein presentation was made possible by support from The Barra Foundation and Unisys.
Special thanks to the Archives of the Institute for Advanced Study, Princeton, New Jersey, USA.
Special thanks to University Archives, Department of Rare Books and Special Collections, Princeton University Library. [Portfolio]
Thanks to Roberta Fedder for German to English translation of letter from Albert Einstein.
Read the Committee on Science and the Arts Report on Albert Einstein’s early life and his Quantum Theory and Theory of Relativity.